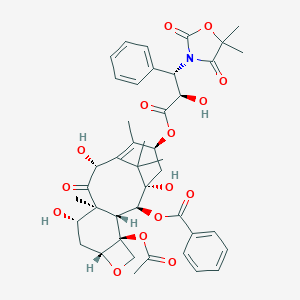
Docetaxel Metabolite M4
概要
説明
Docetaxel Metabolite M4 is a major human metabolite of Docetaxel . It has a molecular formula of C43H49NO15 and a molecular weight of 819.85 .
Synthesis Analysis
Docetaxel, a taxane antitumor agent, was administered to patients by a 1-h intravenous infusion with pharmacokinetic monitoring . The cyclized oxazolidinedione metabolite M4 was most frequently present and was detected in 8 out of 24 patients with maximal concentrations between 0.022 and 0.23 mg/l .Molecular Structure Analysis
The molecular structure of Docetaxel Metabolite M4 is complex, with a molecular formula of C43H49NO15 . More detailed information about its molecular structure may be found in specialized databases or scientific literature .Chemical Reactions Analysis
Docetaxel is metabolized by the CYP3A4/5 into four metabolites: M1, M2, M3, and M4 . The metabolism of Docetaxel to M4 is predicted by logistic regression analysis, with alanine aminotransferase and alkaline phosphatase levels being the strongest predictors .Physical And Chemical Properties Analysis
Docetaxel Metabolite M4 is a neat product with a molecular weight of 819.85 . More specific physical and chemical properties may be found in the product’s safety data sheet or other specialized resources .科学的研究の応用
Interaction with Other Antineoplastic Drugs
Docetaxel Metabolite M4 has been studied for its interaction with other antineoplastic drugs. It has been found that Docetaxel abolishes the hydroxylation of paclitaxel metabolite M4, but is totally inactive on its 6α-hydroxylation . Conversely, paclitaxel significantly reduces the hydroxylation of docetaxel .
Role in Chemotherapy
Docetaxel Metabolite M4 plays a crucial role in chemotherapy. It has been observed that the metabolism of docetaxel by human liver microsomes generates a main docetaxel metabolite . This metabolite is identical to the authentic derivative hydroxylated at the butyl group of the C 13 side chain .
Interaction with Cytochromes P450
The metabolism of Docetaxel Metabolite M4 involves Cytochromes P450. Kinetic measurements and chemical and immunological inhibitions have demonstrated that CYP3A is implicated in the hydroxylation of docetaxel .
Use in Nanoplatforms
Docetaxel Metabolite M4 has been incorporated into nanoplatforms, such as nanofibers and nanoparticles . This advancement offers targeted delivery, controlled release, and improved bioavailability, dramatically reducing systemic toxicity and enhancing patient outcomes .
Role in Regenerative Medicine
The use of Docetaxel Metabolite M4-loaded nanoplatforms has opened new avenues in regenerative medicine by facilitating targeted therapy and cellular regeneration .
Interaction with Other Drugs
The possible interaction among docetaxel, paclitaxel, and drugs which could be associated during chemotherapy has been examined in vitro . Certain drugs such as cisplatin, verapamil, doxorubicin, vinblastine, and vincristine did not markedly modify taxoid metabolism .
作用機序
Safety and Hazards
将来の方向性
特性
IUPAC Name |
[(1S,2S,3R,4S,7R,9S,10S,12R,15S)-4-acetyloxy-15-[(2R,3S)-3-(5,5-dimethyl-2,4-dioxo-1,3-oxazolidin-3-yl)-2-hydroxy-3-phenylpropanoyl]oxy-1,9,12-trihydroxy-10,14,17,17-tetramethyl-11-oxo-6-oxatetracyclo[11.3.1.03,10.04,7]heptadec-13-en-2-yl] benzoate | |
---|---|---|
Source | PubChem | |
URL | https://pubchem.ncbi.nlm.nih.gov | |
Description | Data deposited in or computed by PubChem | |
InChI |
InChI=1S/C43H49NO15/c1-21-25(56-36(51)31(48)29(23-14-10-8-11-15-23)44-37(52)40(5,6)59-38(44)53)19-43(54)34(57-35(50)24-16-12-9-13-17-24)32-41(7,33(49)30(47)28(21)39(43,3)4)26(46)18-27-42(32,20-55-27)58-22(2)45/h8-17,25-27,29-32,34,46-48,54H,18-20H2,1-7H3/t25-,26-,27+,29-,30+,31+,32-,34-,41+,42-,43+/m0/s1 | |
Source | PubChem | |
URL | https://pubchem.ncbi.nlm.nih.gov | |
Description | Data deposited in or computed by PubChem | |
InChI Key |
WZZFVRAJNWWNDL-AYORVWLJSA-N | |
Source | PubChem | |
URL | https://pubchem.ncbi.nlm.nih.gov | |
Description | Data deposited in or computed by PubChem | |
Canonical SMILES |
CC1=C2C(C(=O)C3(C(CC4C(C3C(C(C2(C)C)(CC1OC(=O)C(C(C5=CC=CC=C5)N6C(=O)C(OC6=O)(C)C)O)O)OC(=O)C7=CC=CC=C7)(CO4)OC(=O)C)O)C)O | |
Source | PubChem | |
URL | https://pubchem.ncbi.nlm.nih.gov | |
Description | Data deposited in or computed by PubChem | |
Isomeric SMILES |
CC1=C2[C@H](C(=O)[C@@]3([C@H](C[C@@H]4[C@]([C@H]3[C@@H]([C@@](C2(C)C)(C[C@@H]1OC(=O)[C@@H]([C@H](C5=CC=CC=C5)N6C(=O)C(OC6=O)(C)C)O)O)OC(=O)C7=CC=CC=C7)(CO4)OC(=O)C)O)C)O | |
Source | PubChem | |
URL | https://pubchem.ncbi.nlm.nih.gov | |
Description | Data deposited in or computed by PubChem | |
Molecular Formula |
C43H49NO15 | |
Source | PubChem | |
URL | https://pubchem.ncbi.nlm.nih.gov | |
Description | Data deposited in or computed by PubChem | |
DSSTOX Substance ID |
DTXSID90578107 | |
Record name | (5beta,7beta,10beta,13alpha)-4-(Acetyloxy)-13-{[(2R,3S)-3-(5,5-dimethyl-2,4-dioxo-1,3-oxazolidin-3-yl)-2-hydroxy-3-phenylpropanoyl]oxy}-1,7,10-trihydroxy-9-oxo-5,20-epoxytax-11-en-2-yl benzoate | |
Source | EPA DSSTox | |
URL | https://comptox.epa.gov/dashboard/DTXSID90578107 | |
Description | DSSTox provides a high quality public chemistry resource for supporting improved predictive toxicology. | |
Molecular Weight |
819.8 g/mol | |
Source | PubChem | |
URL | https://pubchem.ncbi.nlm.nih.gov | |
Description | Data deposited in or computed by PubChem | |
Product Name |
Docetaxel Metabolite M4 | |
CAS RN |
157067-34-0 | |
Record name | RPR-104943 | |
Source | ChemIDplus | |
URL | https://pubchem.ncbi.nlm.nih.gov/substance/?source=chemidplus&sourceid=0157067340 | |
Description | ChemIDplus is a free, web search system that provides access to the structure and nomenclature authority files used for the identification of chemical substances cited in National Library of Medicine (NLM) databases, including the TOXNET system. | |
Record name | (5beta,7beta,10beta,13alpha)-4-(Acetyloxy)-13-{[(2R,3S)-3-(5,5-dimethyl-2,4-dioxo-1,3-oxazolidin-3-yl)-2-hydroxy-3-phenylpropanoyl]oxy}-1,7,10-trihydroxy-9-oxo-5,20-epoxytax-11-en-2-yl benzoate | |
Source | EPA DSSTox | |
URL | https://comptox.epa.gov/dashboard/DTXSID90578107 | |
Description | DSSTox provides a high quality public chemistry resource for supporting improved predictive toxicology. | |
Record name | RPR-104943 | |
Source | FDA Global Substance Registration System (GSRS) | |
URL | https://gsrs.ncats.nih.gov/ginas/app/beta/substances/49E4SSQ1AG | |
Description | The FDA Global Substance Registration System (GSRS) enables the efficient and accurate exchange of information on what substances are in regulated products. Instead of relying on names, which vary across regulatory domains, countries, and regions, the GSRS knowledge base makes it possible for substances to be defined by standardized, scientific descriptions. | |
Explanation | Unless otherwise noted, the contents of the FDA website (www.fda.gov), both text and graphics, are not copyrighted. They are in the public domain and may be republished, reprinted and otherwise used freely by anyone without the need to obtain permission from FDA. Credit to the U.S. Food and Drug Administration as the source is appreciated but not required. | |
Retrosynthesis Analysis
AI-Powered Synthesis Planning: Our tool employs the Template_relevance Pistachio, Template_relevance Bkms_metabolic, Template_relevance Pistachio_ringbreaker, Template_relevance Reaxys, Template_relevance Reaxys_biocatalysis model, leveraging a vast database of chemical reactions to predict feasible synthetic routes.
One-Step Synthesis Focus: Specifically designed for one-step synthesis, it provides concise and direct routes for your target compounds, streamlining the synthesis process.
Accurate Predictions: Utilizing the extensive PISTACHIO, BKMS_METABOLIC, PISTACHIO_RINGBREAKER, REAXYS, REAXYS_BIOCATALYSIS database, our tool offers high-accuracy predictions, reflecting the latest in chemical research and data.
Strategy Settings
Precursor scoring | Relevance Heuristic |
---|---|
Min. plausibility | 0.01 |
Model | Template_relevance |
Template Set | Pistachio/Bkms_metabolic/Pistachio_ringbreaker/Reaxys/Reaxys_biocatalysis |
Top-N result to add to graph | 6 |
Feasible Synthetic Routes
試験管内研究製品の免責事項と情報
BenchChemで提示されるすべての記事および製品情報は、情報提供を目的としています。BenchChemで購入可能な製品は、生体外研究のために特別に設計されています。生体外研究は、ラテン語の "in glass" に由来し、生物体の外で行われる実験を指します。これらの製品は医薬品または薬として分類されておらず、FDAから任何の医療状態、病気、または疾患の予防、治療、または治癒のために承認されていません。これらの製品を人間または動物に体内に導入する形態は、法律により厳格に禁止されています。これらのガイドラインに従うことは、研究と実験において法的および倫理的な基準の遵守を確実にするために重要です。