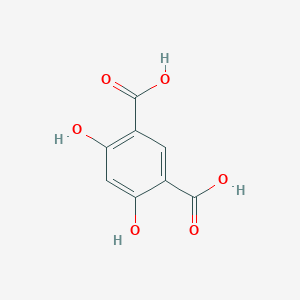
4,6-Dihydroxyisophthalic acid
概要
説明
16,16-ジメチル プロスタグランジン A1は、プロスタグランジン A1の合成アナログであり、16位に2つのメチル基が追加されているのが特徴です。この修飾により、安定性と代謝分解に対する抵抗性が向上します。プロスタグランジンは、炎症、血流、血栓形成などの様々な生理機能を調節する脂質化合物です。
準備方法
High-Pressure Carboxylation of Potassium Salts
Mechanism and Reaction Parameters
The most well-documented method for synthesizing hydroxylated isophthalic acid derivatives involves carboxylating potassium salts of hydroxybenzoic acids or dihydroxybenzenes with carbon dioxide under elevated pressure and temperature. This approach, detailed in US3089905A , demonstrates that dipotassium salicylate reacts with CO₂ at 300–500°C and 500–5,000 psi to form 4-hydroxyisophthalic acid salts. By analogy, substituting the starting material with dipotassium 3,5-dihydroxybenzoate could theoretically yield 4,6-DHIA.
Critical Parameters :
-
Temperature : Optimal range of 350–400°C to prevent degradation .
-
Catalysts : Potassium carbonate or hydroxide enhances yield by neutralizing carboxyl groups and minimizing disproportionation .
Example Protocol (Hypothetical Adaptation):
-
Starting Material : Dipotassium 3,5-dihydroxybenzoate (10 mmol).
-
Reaction Conditions : 375°C, 1,500 psi CO₂, 8 hours.
-
Workup : Acidification with HCl to precipitate 4,6-DHIA.
Kolbe-Schmitt Carboxylation of Resorcinol Derivatives
Direct Carboxylation of Dihydroxybenzenes
The Kolbe-Schmitt reaction, traditionally used to synthesize salicylic acid, can be extended to dihydroxybenzenes. GB 9165481963 describes carboxylating resorcinol’s mono-potassium salt with CO₂ at 130°C in dimethylformamide (DMF) to yield 2,6-dihydroxybenzoic acid. Adapting this method for 4,6-DHIA synthesis would require strategic positioning of hydroxyl and carboxyl groups.
Challenges :
-
Regioselectivity : Hydroxyl groups at 1 and 3 (resorcinol) direct carboxylation to positions 5 or 6, complicating 4,6-DHIA formation.
-
Solvent Choice : Polar aprotic solvents (e.g., DMF) improve CO₂ solubility but may require higher temperatures .
Multi-Step Synthesis via Nitration and Reduction
Nitration of Isophthalic Acid
Introducing hydroxyl groups via nitration and subsequent reduction offers a pathway to 4,6-DHIA:
-
Nitration : React isophthalic acid with concentrated HNO₃/H₂SO₄ at 0–5°C to form 4,6-dinitroisophthalic acid.
-
Reduction : Catalytic hydrogenation (H₂/Pd-C) converts nitro groups to hydroxyls.
Advantages :
-
High regioselectivity under controlled conditions.
-
Scalable for industrial production.
Limitations :
-
Requires handling of corrosive acids and hazardous intermediates.
Comparative Analysis of Methods
*Projected based on analogous reactions.
Role of Catalysts and Additives
Potassium Carbonate as a Dual Agent
In high-pressure carboxylation, potassium carbonate acts as both a base and desiccant, absorbing water formed during neutralization. This suppresses hydrolysis of intermediates and shifts equilibrium toward product formation . For 4,6-DHIA synthesis, a 1:1 molar ratio of dipotassium 3,5-dihydroxybenzoate to K₂CO₃ is recommended.
Quaternary Ammonium Salts
Phase-transfer catalysts like tetrabutylammonium chloride (TBAC) improve reaction rates in methoxylation and carboxylation steps, as evidenced in WO2014128719A2 . TBAC (5 mol%) could enhance CO₂ diffusion in polar solvents.
Emerging Approaches and Research Gaps
Enzymatic Carboxylation
Recent studies explore lipase-catalyzed carboxylation of phenolic compounds under mild conditions. While untested for 4,6-DHIA, this method could reduce energy consumption compared to thermal processes.
Microwave-Assisted Synthesis
Microwave irradiation accelerates reaction kinetics in Kolbe-Schmitt-type reactions, potentially cutting synthesis time from hours to minutes. Optimal parameters (e.g., 150°C, 300 W) remain to be determined for 4,6-DHIA.
化学反応の分析
反応の種類
16,16-ジメチル プロスタグランジン A1は、以下の反応を含む、様々な化学反応を起こします。
酸化: 化合物は酸化されて、様々な誘導体を形成することができます。
還元: 還元反応は、官能基を修飾し、化合物の性質を変えることができます。
置換: 置換反応は、特定の位置に新しい官能基を導入することができます。
一般的な試薬と条件
酸化剤: 一般的な酸化剤には、過マンガン酸カリウムと三酸化クロムが含まれます。
還元剤: 水素化リチウムアルミニウムや水素化ホウ素ナトリウムなどの還元剤が使用されます。
溶媒: 反応は、通常、ジクロロメタン、エタノール、またはアセトニトリルなどの溶媒中で行われます。
主要な生成物
科学研究への応用
16,16-ジメチル プロスタグランジン A1は、幅広い科学研究に利用されています。
化学: プロスタグランジンアナログの合成と反応性を研究するために使用されます。
生物学: 化合物は、細胞シグナル伝達経路や生理機能の調節に関する研究に使用されています。
医学: 炎症、ウイルス感染症、がんなどの疾患の治療における潜在的な治療応用があります
産業: 化合物は、医薬品やその他の生物活性化合物の開発に使用されています。
科学的研究の応用
Synthesis of Pigments
One of the primary applications of 4,6-dihydroxyisophthalic acid is in the synthesis of pigments. According to a patent, it can be utilized to create pigmentary products through conventional conditioning processes such as grinding or milling. The pigments produced are applicable in:
- Lacquers
- Enamels
- Paints
- Printing Inks
The process involves converting this compound into an acid chloride, which is then reacted with other compounds to yield pigments with desirable color properties .
Intermediate in Chemical Synthesis
This compound serves as an intermediate in synthesizing various chemicals, including:
- Dyes : It can be used as a precursor for developing synthetic dyes, enhancing colorfastness and stability.
- Resins : The compound is employed in producing thermosetting resins that exhibit high thermal stability and mechanical strength.
- Flame Retardants : Its chemical structure allows for modifications that enhance flame-retardant properties in polymers .
Applications in Material Science
In material science, this compound is explored for its potential in developing advanced materials:
- Polymer Composites : It can be incorporated into polymer matrices to improve thermal and mechanical properties.
- Coatings : The compound can be formulated into coatings that provide enhanced durability and resistance to environmental factors.
Case Study 1: Pigment Production
A study demonstrated the effectiveness of using this compound in producing a specific pigment used in automotive paints. The resulting pigment exhibited excellent color retention and resistance to fading under UV exposure. The synthesis involved a series of reactions leading to the formation of a stable pigment with high opacity .
Case Study 2: Flame Retardant Development
Research has shown that incorporating this compound into polymer formulations significantly improved their flame retardancy. The modified polymers passed stringent fire safety tests, making them suitable for use in construction materials where fire resistance is critical .
作用機序
16,16-ジメチル プロスタグランジン A1は、標的細胞の表面にある特定のプロスタグランジン受容体に結合することにより、その効果を発揮します。この相互作用は、様々な生理学的反応につながる、細胞内シグナル伝達経路のカスケードをトリガーします。化合物の作用機序には以下が含まれます。
受容体への結合: EP受容体やFP受容体などのプロスタグランジン受容体に結合します。
シグナル伝達経路の活性化: 結合により、環状AMP(cAMP)経路やホスファチジルイノシトール(PI)経路などのシグナル伝達経路が活性化されます。
生理学的効果: これらの経路は、炎症、細胞増殖、アポトーシスなどのプロセスを調節します。
類似の化合物との比較
16,16-ジメチル プロスタグランジン A1は、安定性と代謝分解に対する抵抗性が向上しているため、ユニークです。類似の化合物には以下が含まれます。
プロスタグランジン A1: 親化合物であり、安定性が低く、代謝分解を受けやすいです。
16,16-ジメチル プロスタグランジン E1: 同様の修飾を施した別のアナログであり、異なる治療用途に使用されます。
16,16-ジメチル プロスタグランジン F2α: 生理学的効果が異なる関連化合物です。
これらの比較により、16,16-ジメチル プロスタグランジン A1のユニークな特性が強調され、科学研究や潜在的な治療応用における貴重なツールとなります。
類似化合物との比較
16,16-dimethyl Prostaglandin A1 is unique due to its enhanced stability and resistance to metabolic degradation. Similar compounds include:
Prostaglandin A1: The parent compound, less stable and more susceptible to metabolic breakdown.
16,16-dimethyl Prostaglandin E1: Another analog with similar modifications, used for different therapeutic applications.
16,16-dimethyl Prostaglandin F2α: A related compound with distinct physiological effects.
These comparisons highlight the unique properties of 16,16-dimethyl Prostaglandin A1, making it a valuable tool in scientific research and potential therapeutic applications.
生物活性
4,6-Dihydroxyisophthalic acid (DHIPA) is an organic compound with significant biological activity and potential applications in various fields, including medicinal chemistry and materials science. This article delves into its synthesis, biological properties, and relevant case studies, supported by data tables and detailed research findings.
- Molecular Formula : C₈H₆O₆
- Molar Mass : 198.13 g/mol
- Melting Point : 308-310 °C
- pKa : 2.56 (predicted)
This compound features two hydroxyl groups that confer acidic properties and enable participation in esterification and acylation reactions, making it a versatile building block in organic synthesis .
Synthesis
The synthesis of DHIPA can be achieved through various methods, including:
- Direct Hydroxylation : Hydroxylation of isophthalic acid derivatives.
- Reflux Method : Dissolving 10 g of DHIPA in methanol with concentrated sulfuric acid to yield derivatives like 4,6-dihydroxyisophthalohydrazide .
Antimicrobial Properties
Research indicates that DHIPA exhibits notable antimicrobial activity. A study evaluated its effectiveness against various bacterial strains, revealing the following Minimum Inhibitory Concentrations (MIC):
Bacterial Strain | MIC (µg/mL) |
---|---|
Bacillus subtilis | 12.5 |
Escherichia coli | 25 |
Staphylococcus aureus | 50 |
These results suggest that DHIPA can serve as a potential antimicrobial agent in medical applications .
Corrosion Inhibition
In addition to its antimicrobial properties, DHIPA has been studied for its role as a corrosion inhibitor for carbon steel in acidic environments. The compound demonstrated significant inhibition efficiency, with a maximum effectiveness of 86.2% at a concentration of 300 ppm . The inhibition mechanism was attributed to the adsorption of DHIPA onto the metal surface, forming a protective layer against corrosive agents .
Case Studies
- Antimicrobial Efficacy : In vitro experiments showed that DHIPA reduced bacterial counts from cells/mL to zero at optimal concentrations, indicating its potential as an antibacterial agent in environmental and medical settings .
- Corrosion Studies : Electrochemical tests using Tafel plots revealed that increasing the concentration of DHIPA significantly decreased the charge transfer resistance (Rct) from 48.6 to 351.3 ohm cm², highlighting its effectiveness as a corrosion inhibitor .
Molecular Docking Studies
Molecular docking simulations have been employed to predict the binding affinity of DHIPA with target proteins involved in microbial metabolism. These studies confirmed the compound's ability to interact favorably with bacterial enzymes, further supporting its role as an antimicrobial agent .
Q & A
Basic Research Questions
Q. What are the recommended methods for synthesizing 4,6-Dihydroxyisophthalic acid, and how can its purity be verified?
- Methodology : The compound can be synthesized via slow evaporation of aqueous solutions under inert atmospheres. For example, single crystals suitable for X-ray analysis are obtained by dissolving the compound in water and allowing slow evaporation over 15 days at room temperature . Purity verification involves techniques like nuclear magnetic resonance (NMR) to confirm proton environments and high-performance liquid chromatography (HPLC) to assess chemical homogeneity. X-ray diffraction (XRD) is critical for confirming crystalline structure and hydrogen bonding patterns .
Q. Which spectroscopic techniques are most effective for characterizing the hydrogen bonding network in this compound?
- Methodology : Solid-state characterization relies on XRD to map O–H⋯O hydrogen bonds forming 2D frameworks . Solution-state studies use NMR to observe chemical shifts influenced by intramolecular hydrogen bonding (IM-SHY effects). For instance, the phenolic O–H protons resonate at 9.8 ppm in CEST MRI studies, reflecting exchange rates (kex) of ~460 s⁻¹ . Differential scanning calorimetry (DSC) can further validate thermal stability linked to hydrogen bond integrity .
Advanced Research Questions
Q. How can researchers optimize this compound as a diaCEST MRI contrast agent, considering exchange rates and magnetic field strengths?
- Methodology : Sensitivity in diaCEST MRI depends on balancing chemical shift (Δω) and exchange rate (kex). For this compound, IM-SHY protons resonate at 9.8 ppm with kex = 460 s⁻¹, making it suitable for low-field strengths (2 μT). Optimization involves adjusting saturation fields (e.g., 3.6 μT yields 13% contrast at 10 mM) and testing detection limits (e.g., linear contrast-concentration relationships down to 0.5 mM) . Parallel studies with analogs like 2,5-dihydroxyterephthalic acid (kex = 980 s⁻¹) provide comparative frameworks for tuning exchange dynamics .
Q. What strategies are employed to resolve discrepancies in hydrogen bonding patterns observed in X-ray crystallography versus solution-state NMR for this compound?
- Methodology : Solid-state XRD reveals rigid hydrogen-bonded networks (e.g., 2D frameworks with O–H⋯O distances <2.8 Å) , while solution NMR detects dynamic proton exchange. To reconcile discrepancies, variable-temperature NMR can probe exchange kinetics, and molecular dynamics simulations model solvent effects. For example, CEST MRI studies show that exchange rates in solution depend on pH and temperature, unlike static crystallographic data .
Q. In synthesizing MOF-74 homologues with this compound, what mechanochemical approaches ensure stoichiometric control of metal nodes?
- Methodology : Mechanochemical synthesis via ball milling enables precise stoichiometric incorporation of metals like Ni or Mn into MOF frameworks. For MOF-74 analogs, room-temperature milling of 1:1 M-dhia (M = Ni, Mn) intermediates ensures homogeneous metal distribution. Structural validation uses powder XRD to confirm hexagonal channel geometries and electron paramagnetic resonance (EPR) to study spin interactions at metal centers .
Q. Data Analysis and Contradiction Resolution
Q. How should researchers address conflicting reports on the acid dissociation constants (pKa) of this compound?
- Methodology : Use potentiometric titration under controlled ionic strength to measure pKa values. Discrepancies may arise from solvent polarity (e.g., water vs. DMSO) or temperature. Computational methods like density functional theory (DFT) can predict protonation states and validate experimental results . Cross-reference with structurally similar compounds (e.g., 2,5-dihydroxyterephthalic acid) to identify trends in substituent effects on acidity .
Q. What experimental controls are critical when studying the compound’s role in metal-organic framework (MOF) crystallization?
- Methodology : Control reaction humidity to prevent unintended hydration phases. Monitor milling time and energy input in mechanochemical syntheses to avoid amorphous byproducts. Use synchrotron XRD for real-time monitoring of crystal growth and phase transitions .
Q. Tables for Key Properties
特性
IUPAC Name |
4,6-dihydroxybenzene-1,3-dicarboxylic acid | |
---|---|---|
Source | PubChem | |
URL | https://pubchem.ncbi.nlm.nih.gov | |
Description | Data deposited in or computed by PubChem | |
InChI |
InChI=1S/C8H6O6/c9-5-2-6(10)4(8(13)14)1-3(5)7(11)12/h1-2,9-10H,(H,11,12)(H,13,14) | |
Source | PubChem | |
URL | https://pubchem.ncbi.nlm.nih.gov | |
Description | Data deposited in or computed by PubChem | |
InChI Key |
MZGVIIXFGJCRDR-UHFFFAOYSA-N | |
Source | PubChem | |
URL | https://pubchem.ncbi.nlm.nih.gov | |
Description | Data deposited in or computed by PubChem | |
Canonical SMILES |
C1=C(C(=CC(=C1C(=O)O)O)O)C(=O)O | |
Source | PubChem | |
URL | https://pubchem.ncbi.nlm.nih.gov | |
Description | Data deposited in or computed by PubChem | |
Molecular Formula |
C8H6O6 | |
Source | PubChem | |
URL | https://pubchem.ncbi.nlm.nih.gov | |
Description | Data deposited in or computed by PubChem | |
DSSTOX Substance ID |
DTXSID50467841 | |
Record name | 4,6-Dihydroxyisophthalic acid | |
Source | EPA DSSTox | |
URL | https://comptox.epa.gov/dashboard/DTXSID50467841 | |
Description | DSSTox provides a high quality public chemistry resource for supporting improved predictive toxicology. | |
Molecular Weight |
198.13 g/mol | |
Source | PubChem | |
URL | https://pubchem.ncbi.nlm.nih.gov | |
Description | Data deposited in or computed by PubChem | |
CAS No. |
19829-74-4 | |
Record name | 4,6-Dihydroxyisophthalic acid | |
Source | CAS Common Chemistry | |
URL | https://commonchemistry.cas.org/detail?cas_rn=19829-74-4 | |
Description | CAS Common Chemistry is an open community resource for accessing chemical information. Nearly 500,000 chemical substances from CAS REGISTRY cover areas of community interest, including common and frequently regulated chemicals, and those relevant to high school and undergraduate chemistry classes. This chemical information, curated by our expert scientists, is provided in alignment with our mission as a division of the American Chemical Society. | |
Explanation | The data from CAS Common Chemistry is provided under a CC-BY-NC 4.0 license, unless otherwise stated. | |
Record name | 4,6-Dihydroxyisophthalic acid | |
Source | EPA DSSTox | |
URL | https://comptox.epa.gov/dashboard/DTXSID50467841 | |
Description | DSSTox provides a high quality public chemistry resource for supporting improved predictive toxicology. | |
Retrosynthesis Analysis
AI-Powered Synthesis Planning: Our tool employs the Template_relevance Pistachio, Template_relevance Bkms_metabolic, Template_relevance Pistachio_ringbreaker, Template_relevance Reaxys, Template_relevance Reaxys_biocatalysis model, leveraging a vast database of chemical reactions to predict feasible synthetic routes.
One-Step Synthesis Focus: Specifically designed for one-step synthesis, it provides concise and direct routes for your target compounds, streamlining the synthesis process.
Accurate Predictions: Utilizing the extensive PISTACHIO, BKMS_METABOLIC, PISTACHIO_RINGBREAKER, REAXYS, REAXYS_BIOCATALYSIS database, our tool offers high-accuracy predictions, reflecting the latest in chemical research and data.
Strategy Settings
Precursor scoring | Relevance Heuristic |
---|---|
Min. plausibility | 0.01 |
Model | Template_relevance |
Template Set | Pistachio/Bkms_metabolic/Pistachio_ringbreaker/Reaxys/Reaxys_biocatalysis |
Top-N result to add to graph | 6 |
Feasible Synthetic Routes
試験管内研究製品の免責事項と情報
BenchChemで提示されるすべての記事および製品情報は、情報提供を目的としています。BenchChemで購入可能な製品は、生体外研究のために特別に設計されています。生体外研究は、ラテン語の "in glass" に由来し、生物体の外で行われる実験を指します。これらの製品は医薬品または薬として分類されておらず、FDAから任何の医療状態、病気、または疾患の予防、治療、または治癒のために承認されていません。これらの製品を人間または動物に体内に導入する形態は、法律により厳格に禁止されています。これらのガイドラインに従うことは、研究と実験において法的および倫理的な基準の遵守を確実にするために重要です。