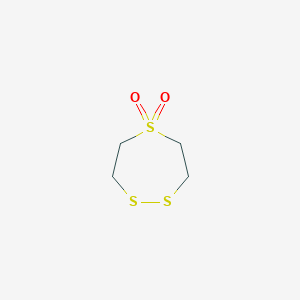
ビス(2-メルカプトエチル)スルホンジスルフィド
概要
説明
Bis(2-mercaptoethyl) sulfone disulfide is a chemical compound with the molecular formula C4H8O2S3. It is known for its ability to reduce disulfides in aqueous solutions, making it a superior substitute for dithiothreitol (DTT) in various biochemical applications . This compound is particularly valued in proteomics research due to its efficiency in reducing disulfide bonds .
科学的研究の応用
Bis(2-mercaptoethyl) sulfone disulfide has a wide range of applications in scientific research:
作用機序
Target of Action
Bis(2-mercaptoethyl) Sulfone Disulfide, also known as BMS, primarily targets disulfide bonds in proteins . These bonds play a crucial role in maintaining the three-dimensional structure of proteins, which is essential for their function.
Mode of Action
BMS interacts with its targets by acting as a reducing agent . It breaks the disulfide bonds in proteins, thereby altering their structure. This change can affect the function of the proteins, leading to various downstream effects.
Biochemical Pathways
The reduction of disulfide bonds by BMS can affect multiple biochemical pathways. For instance, it can influence the folding and stability of proteins, which are critical for their function . The exact pathways affected would depend on the specific proteins targeted by BMS.
Result of Action
The reduction of disulfide bonds by BMS can lead to significant molecular and cellular effects. For example, it has been shown to reduce accessible disulfide bonds in immunoglobulin and trypsinogen under non-denaturing conditions 5 to 7 times faster than DTT at pH 7 . This can potentially alter the function of these proteins, leading to changes at the cellular level.
Action Environment
The action of BMS can be influenced by various environmental factors. For instance, its reducing activity is known to be effective at pH 7 . Therefore, changes in pH could potentially affect its efficacy. Additionally, as a water-soluble compound, its stability and action could be influenced by the hydration status of the environment.
Safety and Hazards
生化学分析
Biochemical Properties
Bis(2-mercaptoethyl) Sulfone Disulfide has been described as a useful compound for the reduction of disulfides in aqueous solutions . It has shown to reduce accessible disulfide bonds in immunoglobulin and trypsinogen under non-denaturing conditions 5 to 7 times faster than DTT at pH 7 . The relatively less accessible disulfide bond in α-chymotrypsinogen A was reduced 2.3 times faster with Bis(2-mercaptoethyl) Sulfone Disulfide than with DTT at pH 7.0 .
Cellular Effects
The cellular effects of Bis(2-mercaptoethyl) Sulfone Disulfide are primarily related to its role in the reduction of disulfide bonds in proteins . By reducing these bonds, it can influence protein structure and function, which can have downstream effects on cell signaling pathways, gene expression, and cellular metabolism .
Molecular Mechanism
The molecular mechanism of action of Bis(2-mercaptoethyl) Sulfone Disulfide involves the reduction of disulfide bonds in proteins . This can lead to changes in protein conformation and activity, which can in turn influence cellular processes .
準備方法
Synthetic Routes and Reaction Conditions: The synthesis of bis(2-mercaptoethyl) sulfone disulfide typically involves the reaction of 2-mercaptoethanol with sulfur dioxide under controlled conditions. The reaction proceeds through the formation of an intermediate sulfone, which is then oxidized to form the disulfide compound .
Industrial Production Methods: In industrial settings, the production of bis(2-mercaptoethyl) sulfone disulfide involves large-scale reactions in reactors designed to handle the specific conditions required for the synthesis. The process includes steps such as purification and crystallization to ensure the compound’s high purity .
Types of Reactions:
Reduction: Bis(2-mercaptoethyl) sulfone disulfide is primarily used as a reducing agent.
Oxidation: The compound can undergo oxidation to form sulfonic acid derivatives under specific conditions.
Common Reagents and Conditions:
Major Products Formed:
類似化合物との比較
Dithiothreitol (DTT): A commonly used reducing agent in biochemical research.
2-Mercaptoethanol: Another reducing agent used in similar applications.
Comparison:
特性
IUPAC Name |
1,2,5-trithiepane 5,5-dioxide | |
---|---|---|
Source | PubChem | |
URL | https://pubchem.ncbi.nlm.nih.gov | |
Description | Data deposited in or computed by PubChem | |
InChI |
InChI=1S/C4H8O2S3/c5-9(6)3-1-7-8-2-4-9/h1-4H2 | |
Source | PubChem | |
URL | https://pubchem.ncbi.nlm.nih.gov | |
Description | Data deposited in or computed by PubChem | |
InChI Key |
IFMXYCMBQCPKAU-UHFFFAOYSA-N | |
Source | PubChem | |
URL | https://pubchem.ncbi.nlm.nih.gov | |
Description | Data deposited in or computed by PubChem | |
Canonical SMILES |
C1CS(=O)(=O)CCSS1 | |
Source | PubChem | |
URL | https://pubchem.ncbi.nlm.nih.gov | |
Description | Data deposited in or computed by PubChem | |
Molecular Formula |
C4H8O2S3 | |
Source | PubChem | |
URL | https://pubchem.ncbi.nlm.nih.gov | |
Description | Data deposited in or computed by PubChem | |
Molecular Weight |
184.3 g/mol | |
Source | PubChem | |
URL | https://pubchem.ncbi.nlm.nih.gov | |
Description | Data deposited in or computed by PubChem | |
Retrosynthesis Analysis
AI-Powered Synthesis Planning: Our tool employs the Template_relevance Pistachio, Template_relevance Bkms_metabolic, Template_relevance Pistachio_ringbreaker, Template_relevance Reaxys, Template_relevance Reaxys_biocatalysis model, leveraging a vast database of chemical reactions to predict feasible synthetic routes.
One-Step Synthesis Focus: Specifically designed for one-step synthesis, it provides concise and direct routes for your target compounds, streamlining the synthesis process.
Accurate Predictions: Utilizing the extensive PISTACHIO, BKMS_METABOLIC, PISTACHIO_RINGBREAKER, REAXYS, REAXYS_BIOCATALYSIS database, our tool offers high-accuracy predictions, reflecting the latest in chemical research and data.
Strategy Settings
Precursor scoring | Relevance Heuristic |
---|---|
Min. plausibility | 0.01 |
Model | Template_relevance |
Template Set | Pistachio/Bkms_metabolic/Pistachio_ringbreaker/Reaxys/Reaxys_biocatalysis |
Top-N result to add to graph | 6 |
Feasible Synthetic Routes
試験管内研究製品の免責事項と情報
BenchChemで提示されるすべての記事および製品情報は、情報提供を目的としています。BenchChemで購入可能な製品は、生体外研究のために特別に設計されています。生体外研究は、ラテン語の "in glass" に由来し、生物体の外で行われる実験を指します。これらの製品は医薬品または薬として分類されておらず、FDAから任何の医療状態、病気、または疾患の予防、治療、または治癒のために承認されていません。これらの製品を人間または動物に体内に導入する形態は、法律により厳格に禁止されています。これらのガイドラインに従うことは、研究と実験において法的および倫理的な基準の遵守を確実にするために重要です。