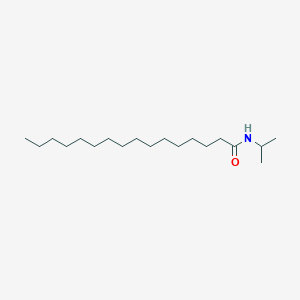
Palmitoylisopropylamide
概要
説明
Palmitoylisopropylamide (PIA) is a synthetic analog of palmitoyl ethanolamide . It incorporates isopropyl amide in place of the native ethanolamide .
Molecular Structure Analysis
The molecular formula of Palmitoylisopropylamide is C19H39NO . Its molecular weight is 297.52 .Physical And Chemical Properties Analysis
Palmitoylisopropylamide is a crystalline solid . It has a solubility of 3 mg/ml in DMF and 20 mg/ml in Ethanol . It should be stored at room temperature .科学的研究の応用
Synthetic Analog of Palmitoyl Ethanolamide
PIA is a synthetic analog of palmitoyl ethanolamide . It incorporates isopropyl amide in place of the native ethanolamide . This modification allows PIA to have similar properties to palmitoyl ethanolamide, but with potentially different biological effects .
Inhibition of Anandamide Transport and Hydrolysis
PIA has been found to inhibit both the transport (re-uptake) of tritiated anandamide into intact cells, as well as the subsequent hydrolysis of anandamide to arachidonate and ethanolamine . This suggests that PIA could be used in research to study the mechanisms of anandamide transport and hydrolysis .
Endocannabinoid Research
PIA does not bind appreciably to either CB1 or CB2 receptors . This makes it a useful tool in endocannabinoid research, as it can be used to study the effects of endocannabinoids without directly activating the CB1 or CB2 receptors .
Lipid Biochemistry
PIA is part of the fatty amides category of lipids . As such, it can be used in lipid biochemistry research to study the properties and functions of fatty amides .
Neuroscience
Given its role in endocannabinoid research and lipid biochemistry, PIA can also be used in neuroscience research . It can help in understanding the role of endocannabinoids and fatty amides in the nervous system .
Cannabinoid Research
PIA’s lack of binding to CB1 or CB2 receptors makes it a valuable tool in cannabinoid research . It can be used to study the effects of cannabinoids without the confounding factor of direct receptor activation .
Safety And Hazards
将来の方向性
While specific future directions for Palmitoylisopropylamide are not detailed in the available resources, one paper suggests that Palmitoylisopropylamide may prove useful as a template for the design of compounds that reduce the cellular accumulation and metabolism of AEA without affecting either CB1 or CB2 receptors .
特性
IUPAC Name |
N-propan-2-ylhexadecanamide | |
---|---|---|
Source | PubChem | |
URL | https://pubchem.ncbi.nlm.nih.gov | |
Description | Data deposited in or computed by PubChem | |
InChI |
InChI=1S/C19H39NO/c1-4-5-6-7-8-9-10-11-12-13-14-15-16-17-19(21)20-18(2)3/h18H,4-17H2,1-3H3,(H,20,21) | |
Source | PubChem | |
URL | https://pubchem.ncbi.nlm.nih.gov | |
Description | Data deposited in or computed by PubChem | |
InChI Key |
TZYVUGCYYQOTQR-UHFFFAOYSA-N | |
Source | PubChem | |
URL | https://pubchem.ncbi.nlm.nih.gov | |
Description | Data deposited in or computed by PubChem | |
Canonical SMILES |
CCCCCCCCCCCCCCCC(=O)NC(C)C | |
Source | PubChem | |
URL | https://pubchem.ncbi.nlm.nih.gov | |
Description | Data deposited in or computed by PubChem | |
Molecular Formula |
C19H39NO | |
Source | PubChem | |
URL | https://pubchem.ncbi.nlm.nih.gov | |
Description | Data deposited in or computed by PubChem | |
Molecular Weight |
297.5 g/mol | |
Source | PubChem | |
URL | https://pubchem.ncbi.nlm.nih.gov | |
Description | Data deposited in or computed by PubChem | |
Product Name |
Palmitoylisopropylamide |
Retrosynthesis Analysis
AI-Powered Synthesis Planning: Our tool employs the Template_relevance Pistachio, Template_relevance Bkms_metabolic, Template_relevance Pistachio_ringbreaker, Template_relevance Reaxys, Template_relevance Reaxys_biocatalysis model, leveraging a vast database of chemical reactions to predict feasible synthetic routes.
One-Step Synthesis Focus: Specifically designed for one-step synthesis, it provides concise and direct routes for your target compounds, streamlining the synthesis process.
Accurate Predictions: Utilizing the extensive PISTACHIO, BKMS_METABOLIC, PISTACHIO_RINGBREAKER, REAXYS, REAXYS_BIOCATALYSIS database, our tool offers high-accuracy predictions, reflecting the latest in chemical research and data.
Strategy Settings
Precursor scoring | Relevance Heuristic |
---|---|
Min. plausibility | 0.01 |
Model | Template_relevance |
Template Set | Pistachio/Bkms_metabolic/Pistachio_ringbreaker/Reaxys/Reaxys_biocatalysis |
Top-N result to add to graph | 6 |
Feasible Synthetic Routes
Q & A
Q1: How does Palmitoylisopropylamide affect intestinal motility?
A: Palmitoylisopropylamide is a selective inhibitor of Fatty Acid Amide Hydrolase (FAAH) []. FAAH is an enzyme responsible for breaking down endocannabinoids like anandamide, which are known to inhibit intestinal motility []. By inhibiting FAAH, Palmitoylisopropylamide prevents the degradation of endocannabinoids, leading to their accumulation and a subsequent decrease in intestinal motility []. This effect was observed to be reduced by the CB1 receptor antagonist rimonabant and in CB1-deficient mice, suggesting that the action of Palmitoylisopropylamide on intestinal motility is mediated through the CB1 receptor pathway [].
Q2: Are there any structural analogs of Palmitoylisopropylamide that exhibit similar effects on FAAH?
A: While the provided abstracts don't explicitly compare the effects of Palmitoylisopropylamide analogs on FAAH, one study mentions that "homologues and analogues of Palmitoylethanolamide" were investigated for their impact on anandamide inactivation []. Palmitoylethanolamide, like Palmitoylisopropylamide, is a substrate of FAAH. This suggests that exploring structural modifications within this class of compounds could yield insights into structure-activity relationships and potentially lead to the discovery of novel FAAH inhibitors with tailored properties.
Q3: What are the potential implications of understanding Palmitoylisopropylamide's interaction with FAAH?
A: Research suggests that FAAH plays a crucial role in regulating intestinal motility, and its dysregulation has been linked to gastrointestinal disorders []. By inhibiting FAAH, compounds like Palmitoylisopropylamide offer a potential therapeutic avenue for managing these conditions. Further investigation into the detailed mechanism of action, structure-activity relationships, and potential off-target effects of Palmitoylisopropylamide and its analogs could contribute to developing new treatments for gastrointestinal motility disorders.
試験管内研究製品の免責事項と情報
BenchChemで提示されるすべての記事および製品情報は、情報提供を目的としています。BenchChemで購入可能な製品は、生体外研究のために特別に設計されています。生体外研究は、ラテン語の "in glass" に由来し、生物体の外で行われる実験を指します。これらの製品は医薬品または薬として分類されておらず、FDAから任何の医療状態、病気、または疾患の予防、治療、または治癒のために承認されていません。これらの製品を人間または動物に体内に導入する形態は、法律により厳格に禁止されています。これらのガイドラインに従うことは、研究と実験において法的および倫理的な基準の遵守を確実にするために重要です。