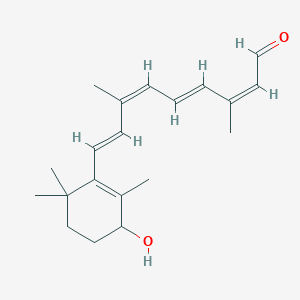
4-Hydroxyretinal
概要
説明
4-Hydroxyretinal is a derivative of retinal, a form of vitamin A. It is characterized by the presence of a hydroxyl group at the fourth position of the cyclohexenyl ring. This compound plays a significant role in various biological processes, including vision, cell differentiation, and growth. It is a natural ligand for several retinoic acid receptors, which are crucial for gene transcription regulation .
準備方法
4-Hydroxyretinal can be synthesized through several methods. One common approach involves the stereoselective synthesis using Horner–Wadsworth–Emmons and Stille cross-coupling reactions. The CBS method of ketone reduction is employed in the enantioface-differentiation step to provide the precursors for the synthesis of the this compound enantiomers . Industrial production methods may involve similar synthetic routes but on a larger scale, ensuring the purity and yield of the compound.
化学反応の分析
4-Hydroxyretinal undergoes various chemical reactions, including:
Oxidation: It can be oxidized to form 4-oxoretinal.
Reduction: Enzymatic reduction by alcohol dehydrogenase can convert it to 4-hydroxyretinol.
Substitution: The hydroxyl group can participate in substitution reactions under specific conditions.
Common reagents used in these reactions include oxidizing agents like potassium permanganate and reducing agents like sodium borohydride. The major products formed from these reactions include 4-oxoretinal and 4-hydroxyretinol .
科学的研究の応用
4-Hydroxyretinal has numerous applications in scientific research:
Chemistry: It is used as a precursor in the synthesis of other retinoids.
Biology: It plays a role in the study of visual processes and cell differentiation.
Medicine: It is investigated for its potential in treating skin disorders and certain types of cancer.
Industry: It is used in the formulation of cosmetic products due to its skin-regenerative properties.
作用機序
4-Hydroxyretinal exerts its effects by binding to retinoic acid receptors, which are ligand-dependent transcription factors. Upon binding, it activates these receptors, leading to the transcription of genes involved in cell growth, differentiation, and apoptosis. The molecular targets include various genes regulated by retinoic acid receptors, and the pathways involved are crucial for maintaining cellular homeostasis .
類似化合物との比較
4-Hydroxyretinal is unique compared to other retinoids due to its specific hydroxylation at the fourth position. Similar compounds include:
Retinal: The parent compound without the hydroxyl group.
4-Oxoretinal: The oxidized form of this compound.
4-Hydroxyretinoic acid: Another hydroxylated derivative with distinct biological activities.
The uniqueness of this compound lies in its specific interaction with retinoic acid receptors and its role in various biological processes .
生物活性
4-Hydroxyretinal, also referred to as vitamin A4, is a chromophore that plays a significant role in the visual systems of various organisms, particularly in arthropods and some vertebrates. Its biological activity is closely related to its structural properties and its interaction with opsins, the proteins responsible for phototransduction in visual processes.
Chemical Structure and Properties
This compound is a derivative of retinal, characterized by the presence of a hydroxyl group at the 4-position of the β-ionone ring. This modification influences its optical properties and binding affinity to opsins. The general structure can be represented as follows:
The presence of the hydroxyl group alters the chromophore's spectral properties, allowing it to absorb light at different wavelengths compared to its counterparts like all-trans-retinal and 11-cis-retinal.
Biological Functions
Visual Pigment Formation
In various species, including crustaceans and certain insects, this compound serves as a chromophore that forms visual pigments when bound to opsins. This binding is crucial for the maturation of visual pigments, which are essential for light detection and phototransduction. Studies have shown that this compound can effectively restore phototransduction in rod photoreceptors exposed to bleached conditions, indicating its potential as a functional substitute for other retinal forms .
Spectral Sensitivity
The spectral sensitivity of photoreceptors utilizing this compound differs from those using other forms of retinal. For instance, in studies involving Chlamydomonas reinhardtii, it was observed that visual responses were restored with various retinal analogs, including this compound, demonstrating its efficacy in inducing phototaxis . The absorption spectrum of this compound typically peaks around 500 nm, which is optimal for certain aquatic environments where these organisms thrive.
Case Study: Chromophore Functionality in Arthropods
Research indicates that arthropods utilize multiple forms of retinal, including this compound. A study highlighted how this compound contributes to the unique visual capabilities of crustaceans by enabling them to perceive a broader spectrum of light . The enzymatic conversion processes involved in synthesizing this chromophore were elucidated using recombinant NinaB proteins from Galleria mellonella, which demonstrated the ability to catalyze oxidative cleavage and isomerization necessary for producing active chromophores .
Fluorescence Properties
Recent studies have explored the fluorescence characteristics of retinal derivatives, including this compound. An increase in fluorescence quantum yields was noted when transitioning from wild-type microbial rhodopsins to variants with modified counterions. This suggests that structural changes related to the hydroxyl group significantly enhance light absorption and retention properties .
Comparative Analysis of Retinal Derivatives
Retinal Type | Absorption Peak (nm) | Biological Role | Organisms Found In |
---|---|---|---|
All-trans-retinal | ~498 | Primary chromophore in vertebrates | Vertebrates |
11-cis-retinal | ~498 | Key role in vertebrate vision | Vertebrates |
3-hydroxyretinal | ~500 | Chromophore in insect vision | Insects |
This compound | ~500 | Chromophore for specific crustacean vision | Crustaceans, some vertebrates |
特性
IUPAC Name |
(2E,4E,6E,8E)-9-(3-hydroxy-2,6,6-trimethylcyclohexen-1-yl)-3,7-dimethylnona-2,4,6,8-tetraenal | |
---|---|---|
Source | PubChem | |
URL | https://pubchem.ncbi.nlm.nih.gov | |
Description | Data deposited in or computed by PubChem | |
InChI |
InChI=1S/C20H28O2/c1-15(7-6-8-16(2)12-14-21)9-10-18-17(3)19(22)11-13-20(18,4)5/h6-10,12,14,19,22H,11,13H2,1-5H3/b8-6+,10-9+,15-7+,16-12+ | |
Source | PubChem | |
URL | https://pubchem.ncbi.nlm.nih.gov | |
Description | Data deposited in or computed by PubChem | |
InChI Key |
CYVVUYORRQQAQE-RMWYGNQTSA-N | |
Source | PubChem | |
URL | https://pubchem.ncbi.nlm.nih.gov | |
Description | Data deposited in or computed by PubChem | |
Canonical SMILES |
CC1=C(C(CCC1O)(C)C)C=CC(=CC=CC(=CC=O)C)C | |
Source | PubChem | |
URL | https://pubchem.ncbi.nlm.nih.gov | |
Description | Data deposited in or computed by PubChem | |
Isomeric SMILES |
CC1=C(C(CCC1O)(C)C)/C=C/C(=C/C=C/C(=C/C=O)/C)/C | |
Source | PubChem | |
URL | https://pubchem.ncbi.nlm.nih.gov | |
Description | Data deposited in or computed by PubChem | |
Molecular Formula |
C20H28O2 | |
Source | PubChem | |
URL | https://pubchem.ncbi.nlm.nih.gov | |
Description | Data deposited in or computed by PubChem | |
Molecular Weight |
300.4 g/mol | |
Source | PubChem | |
URL | https://pubchem.ncbi.nlm.nih.gov | |
Description | Data deposited in or computed by PubChem | |
Retrosynthesis Analysis
AI-Powered Synthesis Planning: Our tool employs the Template_relevance Pistachio, Template_relevance Bkms_metabolic, Template_relevance Pistachio_ringbreaker, Template_relevance Reaxys, Template_relevance Reaxys_biocatalysis model, leveraging a vast database of chemical reactions to predict feasible synthetic routes.
One-Step Synthesis Focus: Specifically designed for one-step synthesis, it provides concise and direct routes for your target compounds, streamlining the synthesis process.
Accurate Predictions: Utilizing the extensive PISTACHIO, BKMS_METABOLIC, PISTACHIO_RINGBREAKER, REAXYS, REAXYS_BIOCATALYSIS database, our tool offers high-accuracy predictions, reflecting the latest in chemical research and data.
Strategy Settings
Precursor scoring | Relevance Heuristic |
---|---|
Min. plausibility | 0.01 |
Model | Template_relevance |
Template Set | Pistachio/Bkms_metabolic/Pistachio_ringbreaker/Reaxys/Reaxys_biocatalysis |
Top-N result to add to graph | 6 |
Feasible Synthetic Routes
試験管内研究製品の免責事項と情報
BenchChemで提示されるすべての記事および製品情報は、情報提供を目的としています。BenchChemで購入可能な製品は、生体外研究のために特別に設計されています。生体外研究は、ラテン語の "in glass" に由来し、生物体の外で行われる実験を指します。これらの製品は医薬品または薬として分類されておらず、FDAから任何の医療状態、病気、または疾患の予防、治療、または治癒のために承認されていません。これらの製品を人間または動物に体内に導入する形態は、法律により厳格に禁止されています。これらのガイドラインに従うことは、研究と実験において法的および倫理的な基準の遵守を確実にするために重要です。