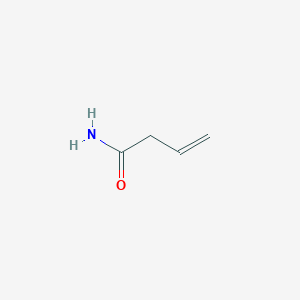
3-Butenamide
概要
説明
It is a white to off-white solid that is slightly soluble in chloroform and methanol . This compound is used in various scientific research applications due to its unique chemical properties.
準備方法
3-Butenamide can be synthesized through several methods. One common synthetic route involves the reaction of butenal with cyanamide. The reaction is typically carried out in a solvent such as methanol at elevated temperatures. The product is then purified through crystallization . Industrial production methods may involve similar synthetic routes but on a larger scale, with optimizations for yield and purity.
化学反応の分析
3-Butenamide undergoes various chemical reactions, including:
Oxidation: It can be oxidized to form corresponding carboxylic acids.
Reduction: It can be reduced to form amines.
Substitution: It can undergo substitution reactions where the amide group is replaced by other functional groups.
Common reagents used in these reactions include oxidizing agents like potassium permanganate for oxidation, reducing agents like lithium aluminum hydride for reduction, and various nucleophiles for substitution reactions. The major products formed depend on the specific reaction conditions and reagents used .
科学的研究の応用
Medicinal Chemistry
1. Potential Therapeutic Applications
3-Butenamide has been studied for its potential therapeutic uses. Research indicates that derivatives of this compound can exhibit biological activities that may be beneficial in treating various conditions:
- Anti-cancer Activity : Certain derivatives have shown promise in inhibiting tumor growth by interfering with cancer cell metabolism and proliferation.
- Neurological Research : Compounds related to this compound have been investigated for their effects on neurotransmitter systems, potentially offering avenues for treating neurodegenerative diseases.
Case Study: Anti-Cancer Properties
A study demonstrated that a derivative of this compound significantly inhibited the growth of specific cancer cell lines by inducing apoptosis (programmed cell death). This finding suggests that further development could lead to new cancer therapies.
Agriculture
2. Agricultural Applications
In agriculture, this compound and its derivatives are being explored as potential agrochemicals:
- Herbicides : Some studies suggest that this compound can act as a herbicide, effectively controlling weed growth without harming crops.
- Plant Growth Regulators : Research indicates that compounds based on this compound may enhance plant growth and yield by modulating hormonal pathways.
Data Table: Herbicidal Activity of this compound Derivatives
Compound Name | Activity Type | Effectiveness (%) | Reference |
---|---|---|---|
2-Methyl-3-butenamide | Herbicide | 85 | |
N-(2-Methylphenyl)-3-butenamide | Growth Regulator | 78 |
Materials Science
3. Industrial Applications
In materials science, this compound is being investigated for its role in synthesizing advanced materials:
- Polymer Production : The compound can be used as a monomer in the production of polymers with specific properties, such as flexibility and thermal stability.
- Coatings and Adhesives : Its chemical structure allows for the formulation of coatings and adhesives that exhibit improved adhesion properties.
作用機序
The mechanism of action of 3-Butenamide involves its interaction with various molecular targets. It can act as a nucleophile in chemical reactions, participating in the formation of new chemical bonds. The specific pathways involved depend on the context of its use, such as in polymerization reactions or as an intermediate in drug synthesis .
類似化合物との比較
3-Butenamide can be compared with other similar compounds such as:
Butanamide: Similar in structure but lacks the double bond present in this compound.
Acrylamide: Contains a similar amide group but with a different carbon chain structure.
Methacrylamide: Similar to acrylamide but with an additional methyl group.
The uniqueness of this compound lies in its double bond, which imparts different reactivity and properties compared to these similar compounds .
生物活性
3-Butenamide, a compound with the molecular formula C₄H₇NO, is recognized for its diverse biological activities. This article explores its pharmacological properties, mechanisms of action, and potential therapeutic applications based on recent research findings.
Molecular Structure:
- Formula: C₄H₇NO
- Molecular Weight: 85.11 g/mol
Structural Characteristics:
The compound features a butenamide backbone, which contributes to its reactivity and biological interactions. The presence of the amide functional group enhances its solubility in biological systems.
Biological Activities
This compound exhibits several notable biological activities, including:
- Anticancer Activity: Research indicates that this compound derivatives can inhibit cancer cell proliferation. For instance, studies have shown that certain analogs of this compound display significant cytotoxic effects against various cancer cell lines by inducing apoptosis and inhibiting cell cycle progression .
- Neuroprotective Effects: The compound has been investigated for its neuroprotective properties. It has been shown to modulate neurotransmitter levels and reduce oxidative stress in neuronal cells, which may have implications for treating neurodegenerative diseases .
- Anti-inflammatory Properties: this compound has demonstrated the ability to reduce inflammation in various models. It inhibits the production of pro-inflammatory cytokines and may be beneficial in conditions characterized by chronic inflammation .
The biological activity of this compound is mediated through several mechanisms:
- Inhibition of Enzymatic Activity: Some studies suggest that this compound can inhibit specific enzymes involved in cancer progression and inflammatory pathways, thereby exerting its therapeutic effects .
- Modulation of Signaling Pathways: The compound appears to influence key signaling pathways associated with cell survival and apoptosis, such as the MAPK/ERK pathway, which is critical in cancer biology .
- Antioxidant Activity: By scavenging free radicals and enhancing the activity of endogenous antioxidant enzymes, this compound may protect against oxidative damage in cells .
Case Studies and Research Findings
A summary of significant research findings regarding the biological activity of this compound is presented in the table below:
特性
IUPAC Name |
but-3-enamide | |
---|---|---|
Source | PubChem | |
URL | https://pubchem.ncbi.nlm.nih.gov | |
Description | Data deposited in or computed by PubChem | |
InChI |
InChI=1S/C4H7NO/c1-2-3-4(5)6/h2H,1,3H2,(H2,5,6) | |
Source | PubChem | |
URL | https://pubchem.ncbi.nlm.nih.gov | |
Description | Data deposited in or computed by PubChem | |
InChI Key |
ABBZJHFBQXYTLU-UHFFFAOYSA-N | |
Source | PubChem | |
URL | https://pubchem.ncbi.nlm.nih.gov | |
Description | Data deposited in or computed by PubChem | |
Canonical SMILES |
C=CCC(=O)N | |
Source | PubChem | |
URL | https://pubchem.ncbi.nlm.nih.gov | |
Description | Data deposited in or computed by PubChem | |
Molecular Formula |
C4H7NO | |
Source | PubChem | |
URL | https://pubchem.ncbi.nlm.nih.gov | |
Description | Data deposited in or computed by PubChem | |
DSSTOX Substance ID |
DTXSID80337280 | |
Record name | 3-Butenamide | |
Source | EPA DSSTox | |
URL | https://comptox.epa.gov/dashboard/DTXSID80337280 | |
Description | DSSTox provides a high quality public chemistry resource for supporting improved predictive toxicology. | |
Molecular Weight |
85.10 g/mol | |
Source | PubChem | |
URL | https://pubchem.ncbi.nlm.nih.gov | |
Description | Data deposited in or computed by PubChem | |
CAS No. |
28446-58-4 | |
Record name | 3-Butenamide | |
Source | EPA DSSTox | |
URL | https://comptox.epa.gov/dashboard/DTXSID80337280 | |
Description | DSSTox provides a high quality public chemistry resource for supporting improved predictive toxicology. | |
Synthesis routes and methods
Procedure details
Retrosynthesis Analysis
AI-Powered Synthesis Planning: Our tool employs the Template_relevance Pistachio, Template_relevance Bkms_metabolic, Template_relevance Pistachio_ringbreaker, Template_relevance Reaxys, Template_relevance Reaxys_biocatalysis model, leveraging a vast database of chemical reactions to predict feasible synthetic routes.
One-Step Synthesis Focus: Specifically designed for one-step synthesis, it provides concise and direct routes for your target compounds, streamlining the synthesis process.
Accurate Predictions: Utilizing the extensive PISTACHIO, BKMS_METABOLIC, PISTACHIO_RINGBREAKER, REAXYS, REAXYS_BIOCATALYSIS database, our tool offers high-accuracy predictions, reflecting the latest in chemical research and data.
Strategy Settings
Precursor scoring | Relevance Heuristic |
---|---|
Min. plausibility | 0.01 |
Model | Template_relevance |
Template Set | Pistachio/Bkms_metabolic/Pistachio_ringbreaker/Reaxys/Reaxys_biocatalysis |
Top-N result to add to graph | 6 |
Feasible Synthetic Routes
Q1: What is the molecular formula and weight of 3-butenamide?
A1: this compound has the molecular formula C4H7NO and a molecular weight of 85.106 g/mol.
Q2: What are the typical spectroscopic characteristics of this compound?
A2: While specific spectroscopic data is limited in the provided research, this compound and its derivatives are commonly characterized using techniques like 1H NMR, 13C NMR, IR spectroscopy, and mass spectrometry. [] These methods help identify key functional groups and structural features.
Q3: Are there alternative synthetic routes to access this compound?
A3: Yes, besides the classical synthesis, a new method for obtaining manganese(III) complexes with this compound derivatives involves electrochemical oxidation of a manganese anode in the presence of the ligand. []
Q4: What is unique about the ring-opening reaction of cyclobutenediones with lithium amides?
A5: Unlike organolithiums that add to the carbonyl group, lithium amides induce ring opening of cyclobutenediones through enone cleavage via O- to C-lithium transfer. This pathway provides access to 2-oxobut-3-enamides and tetrasubstituted furans. []
Q5: Can this compound derivatives undergo cyclization reactions?
A6: Yes, N-aryl-3-butenamides can undergo a silver trifluoromethylthioate (AgSCF3)-mediated tandem trifluoromethylthiolation and cyclization. This reaction shows divergent reactivity, yielding either CF3S-substituted 3,4-dihydroquinolin-2-ones or azaspiro[4,5]dienones depending on the reaction conditions. []
Q6: How does this compound behave under pyrolysis conditions?
A7: Computational studies using Density Functional Theory (DFT) suggest that this compound can form precursors to nitrogen oxides (NOx) and undergo cyclization during pyrolysis. []
Q7: What are some potential applications of this compound derivatives in medicinal chemistry?
A8: Derivatives of this compound have shown potential as specific bradycardic agents. For instance, (E)-N-[3-[N'-[2-(3,4-dimethoxyphenyl)ethyl]-N'-methylamino] propyl]-4-[3,4-(methylenedioxy)phenyl]-3-butenamide displayed potent bradycardic activity in isolated guinea pig right atria and anesthetized dogs. []
Q8: How do this compound derivatives interact with biological systems in the context of HIV?
A9: A novel HIV integrase inhibitor, (Z)-4-[5-(2,6-difluorobenzyl)-1-(2-fluorobenzyl)-2-oxo-1,2-dihydropyridin-3-yl]-4-hydroxy-2-oxo-N-(2-oxopyrrolidin-1-yl)but-3-enamide, has shown potent anti-HIV activity against various HIV isolates. This compound exhibits a dual mechanism of action, targeting both HIV and multidrug-resistant tuberculosis (MDR-TB). [, ]
Q9: What is the metabolic fate of 3-methyl-4-phenyl-3-butenamide?
A10: While specific details on its metabolism are limited in the provided research, 3-methyl-4-phenyl-3-butenamide, a known hypolipidemic compound, undergoes metabolic transformations in biological systems. [] Further research is needed to fully elucidate its metabolic pathways.
試験管内研究製品の免責事項と情報
BenchChemで提示されるすべての記事および製品情報は、情報提供を目的としています。BenchChemで購入可能な製品は、生体外研究のために特別に設計されています。生体外研究は、ラテン語の "in glass" に由来し、生物体の外で行われる実験を指します。これらの製品は医薬品または薬として分類されておらず、FDAから任何の医療状態、病気、または疾患の予防、治療、または治癒のために承認されていません。これらの製品を人間または動物に体内に導入する形態は、法律により厳格に禁止されています。これらのガイドラインに従うことは、研究と実験において法的および倫理的な基準の遵守を確実にするために重要です。