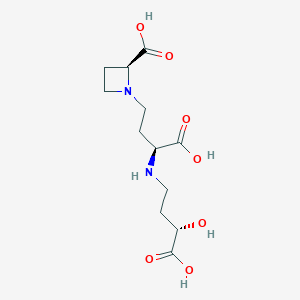
2'-デオキシムギネ酸
概要
説明
2’-Deoxymugineic acid: is a naturally occurring phytosiderophore primarily found in graminaceous plants. It plays a crucial role in the uptake of iron from the soil, particularly in alkaline conditions where iron solubility is low. This compound is a member of the mugineic acid family and is synthesized from methionine via nicotianamine. Its primary function is to chelate iron and facilitate its transport into plant roots, thereby aiding in iron homeostasis.
科学的研究の応用
Chemistry: 2’-Deoxymugineic acid is studied for its role in iron chelation and transport, which has implications for developing new iron fertilizers and improving crop yields in iron-deficient soils .
Biology: Research focuses on understanding the biosynthesis and regulation of 2’-deoxymugineic acid in plants, which can lead to the development of genetically modified crops with enhanced iron uptake capabilities .
Medicine: While direct medical applications are limited, the study of 2’-deoxymugineic acid and its analogs can contribute to the development of new iron chelators for treating iron overload disorders .
生化学分析
Biochemical Properties
2’-Deoxymugineic acid plays a crucial role in biochemical reactions, particularly those involving iron. It interacts with various enzymes and proteins to facilitate iron uptake and transport in plants . For instance, it is involved in the biosynthesis of mugineic acid family phytosiderophores, a process that requires the action of enzymes such as nicotianamine synthase (NAS), nicotianamine aminotransferase (NAAT), and 2’-deoxymugineic acid synthase (DMAS) .
Cellular Effects
2’-Deoxymugineic acid has significant effects on various types of cells and cellular processes. It influences cell function by improving iron nutrition, which is essential for processes like photosynthesis, respiration, and chlorophyll biosynthesis . It also impacts cell signaling pathways, gene expression, and cellular metabolism, primarily through its role in iron homeostasis .
Molecular Mechanism
The molecular mechanism of 2’-Deoxymugineic acid primarily involves its role as a chelator for iron. It forms complexes with iron in the soil, which are then taken up by plant cells . This process involves binding interactions with biomolecules, activation of enzymes involved in iron uptake and transport, and changes in gene expression related to iron homeostasis .
Temporal Effects in Laboratory Settings
The effects of 2’-Deoxymugineic acid can change over time in laboratory settings. While specific studies detailing the temporal effects of 2’-Deoxymugineic acid are limited, it is known that the compound plays a crucial role in the long-term health and development of plants by ensuring adequate iron nutrition .
Dosage Effects in Animal Models
Currently, there is limited information available on the effects of 2’-Deoxymugineic acid dosage in animal models. Most research has focused on its role in plants, particularly in relation to iron uptake and transport .
Metabolic Pathways
2’-Deoxymugineic acid is involved in the metabolic pathway for the biosynthesis of mugineic acid family phytosiderophores . This pathway involves several enzymes, including NAS, NAAT, and DMAS . These enzymes catalyze reactions that convert nicotianamine into 2’-deoxymugineic acid, which is then used to chelate iron .
Transport and Distribution
2’-Deoxymugineic acid is transported and distributed within cells and tissues through specific transporters. For instance, in maize, transporter of MAs (TOM) and efflux transporter of NA (ENA) are involved in the transport of 2’-Deoxymugineic acid .
Subcellular Localization
The subcellular localization of 2’-Deoxymugineic acid is likely to be in the cytoplasm, as suggested by the localization of enzymes involved in its biosynthesis
準備方法
Synthetic Routes and Reaction Conditions: The synthesis of 2’-deoxymugineic acid involves several steps starting from methionine. The key steps include the formation of nicotianamine, followed by its conversion to 2’-deoxymugineic acid through a series of enzymatic reactions. The enzymes involved are nicotianamine synthase, nicotianamine aminotransferase, and a reductase. The final step involves the action of 2’-deoxymugineic-acid 2’-dioxygenase, which converts 2’-deoxymugineic acid to mugineic acid .
Industrial Production Methods: Industrial production of 2’-deoxymugineic acid is not well-documented, as it is primarily studied in the context of plant biology and agriculture. advances in biotechnology and synthetic biology may enable large-scale production through microbial fermentation or plant-based expression systems.
化学反応の分析
Types of Reactions: 2’-Deoxymugineic acid undergoes several types of chemical reactions, including:
Oxidation: Catalyzed by 2’-deoxymugineic-acid 2’-dioxygenase, converting it to mugineic acid.
Chelation: Forms stable complexes with iron, facilitating its uptake by plants.
Common Reagents and Conditions:
Oxidation: Requires 2-oxoglutarate and molecular oxygen as co-substrates.
Chelation: Typically occurs in the rhizosphere under natural soil conditions.
Major Products:
Oxidation: Mugineic acid, succinate, and carbon dioxide.
Chelation: Iron-2’-deoxymugineic acid complex.
作用機序
2’-Deoxymugineic acid exerts its effects primarily through iron chelation. It binds to iron in the soil, forming a stable complex that is readily taken up by plant roots. The iron-2’-deoxymugineic acid complex is transported into the plant cells, where iron is released and utilized for various metabolic processes. The molecular targets include iron transporters and receptors in the plant root cells .
類似化合物との比較
Mugineic acid: Another member of the mugineic acid family, also involved in iron chelation and transport.
Nicotianamine: A precursor in the biosynthesis of 2’-deoxymugineic acid, also functions as an iron chelator.
Uniqueness: 2’-Deoxymugineic acid is unique due to its specific role in the initial steps of iron chelation and its conversion to mugineic acid. Its ability to function effectively in alkaline soils makes it particularly valuable for improving iron uptake in crops grown in such conditions .
特性
IUPAC Name |
(2S)-1-[(3S)-3-carboxy-3-[[(3S)-3-carboxy-3-hydroxypropyl]amino]propyl]azetidine-2-carboxylic acid | |
---|---|---|
Source | PubChem | |
URL | https://pubchem.ncbi.nlm.nih.gov | |
Description | Data deposited in or computed by PubChem | |
InChI |
InChI=1S/C12H20N2O7/c15-9(12(20)21)1-4-13-7(10(16)17)2-5-14-6-3-8(14)11(18)19/h7-9,13,15H,1-6H2,(H,16,17)(H,18,19)(H,20,21)/t7-,8-,9-/m0/s1 | |
Source | PubChem | |
URL | https://pubchem.ncbi.nlm.nih.gov | |
Description | Data deposited in or computed by PubChem | |
InChI Key |
CUZKLRTTYZOCSD-CIUDSAMLSA-N | |
Source | PubChem | |
URL | https://pubchem.ncbi.nlm.nih.gov | |
Description | Data deposited in or computed by PubChem | |
Canonical SMILES |
C1CN(C1C(=O)O)CCC(C(=O)O)NCCC(C(=O)O)O | |
Source | PubChem | |
URL | https://pubchem.ncbi.nlm.nih.gov | |
Description | Data deposited in or computed by PubChem | |
Isomeric SMILES |
C1CN([C@@H]1C(=O)O)CC[C@@H](C(=O)O)NCC[C@@H](C(=O)O)O | |
Source | PubChem | |
URL | https://pubchem.ncbi.nlm.nih.gov | |
Description | Data deposited in or computed by PubChem | |
Molecular Formula |
C12H20N2O7 | |
Source | PubChem | |
URL | https://pubchem.ncbi.nlm.nih.gov | |
Description | Data deposited in or computed by PubChem | |
DSSTOX Substance ID |
DTXSID50995715 | |
Record name | 1-{3-Carboxy-3-[(3-carboxy-3-hydroxypropyl)amino]propyl}azetidine-2-carboxylic acid | |
Source | EPA DSSTox | |
URL | https://comptox.epa.gov/dashboard/DTXSID50995715 | |
Description | DSSTox provides a high quality public chemistry resource for supporting improved predictive toxicology. | |
Molecular Weight |
304.30 g/mol | |
Source | PubChem | |
URL | https://pubchem.ncbi.nlm.nih.gov | |
Description | Data deposited in or computed by PubChem | |
CAS No. |
74235-24-8 | |
Record name | 2′-Deoxymugineic acid | |
Source | CAS Common Chemistry | |
URL | https://commonchemistry.cas.org/detail?cas_rn=74235-24-8 | |
Description | CAS Common Chemistry is an open community resource for accessing chemical information. Nearly 500,000 chemical substances from CAS REGISTRY cover areas of community interest, including common and frequently regulated chemicals, and those relevant to high school and undergraduate chemistry classes. This chemical information, curated by our expert scientists, is provided in alignment with our mission as a division of the American Chemical Society. | |
Explanation | The data from CAS Common Chemistry is provided under a CC-BY-NC 4.0 license, unless otherwise stated. | |
Record name | 2'-Deoxymugineic acid | |
Source | ChemIDplus | |
URL | https://pubchem.ncbi.nlm.nih.gov/substance/?source=chemidplus&sourceid=0074235248 | |
Description | ChemIDplus is a free, web search system that provides access to the structure and nomenclature authority files used for the identification of chemical substances cited in National Library of Medicine (NLM) databases, including the TOXNET system. | |
Record name | 1-{3-Carboxy-3-[(3-carboxy-3-hydroxypropyl)amino]propyl}azetidine-2-carboxylic acid | |
Source | EPA DSSTox | |
URL | https://comptox.epa.gov/dashboard/DTXSID50995715 | |
Description | DSSTox provides a high quality public chemistry resource for supporting improved predictive toxicology. | |
Retrosynthesis Analysis
AI-Powered Synthesis Planning: Our tool employs the Template_relevance Pistachio, Template_relevance Bkms_metabolic, Template_relevance Pistachio_ringbreaker, Template_relevance Reaxys, Template_relevance Reaxys_biocatalysis model, leveraging a vast database of chemical reactions to predict feasible synthetic routes.
One-Step Synthesis Focus: Specifically designed for one-step synthesis, it provides concise and direct routes for your target compounds, streamlining the synthesis process.
Accurate Predictions: Utilizing the extensive PISTACHIO, BKMS_METABOLIC, PISTACHIO_RINGBREAKER, REAXYS, REAXYS_BIOCATALYSIS database, our tool offers high-accuracy predictions, reflecting the latest in chemical research and data.
Strategy Settings
Precursor scoring | Relevance Heuristic |
---|---|
Min. plausibility | 0.01 |
Model | Template_relevance |
Template Set | Pistachio/Bkms_metabolic/Pistachio_ringbreaker/Reaxys/Reaxys_biocatalysis |
Top-N result to add to graph | 6 |
Feasible Synthetic Routes
Q1: How does DMA interact with iron and what are the downstream effects in plants?
A1: DMA acts as a chelating agent, binding to Fe(III) with high affinity in the rhizosphere []. This DMA-Fe(III) complex is then recognized and transported into root cells via specific transporters like HvYS1 in barley []. This efficient uptake system enables graminaceous plants, particularly in alkaline soils where iron availability is limited, to acquire iron essential for growth and development [].
Q2: What is the molecular formula and weight of DMA?
A2: The molecular formula of DMA is C12H20N2O8, and its molecular weight is 320.3 g/mol [].
Q3: What spectroscopic data is available for characterizing DMA?
A3: Techniques such as nuclear magnetic resonance (NMR) and mass spectrometry (MS) are commonly employed to characterize DMA [, , ].
Q4: Does DMA exhibit any catalytic properties itself?
A4: While DMA itself is not known to possess catalytic properties, its role in iron acquisition indirectly influences numerous enzymatic processes within plants, as iron is a crucial cofactor for many enzymes.
Q5: Have there been any computational studies on DMA and its interactions?
A5: Yes, computational techniques like nano-electrospray ionization Fourier transform ion cyclotron resonance mass spectrometry (nano-ESI-FTICRMS) have been used to analyze DMA, its metal complexes with Fe(II) and Fe(III), and its interactions with other molecules like nicotianamine [].
Q6: How do structural modifications of DMA affect its iron-chelating activity?
A6: Structural modifications can significantly impact DMA's activity. For example, the addition of a hydroxyl group at the 2' position, forming 2'-hydroxyavenic acid A (HAVA), has been shown to maintain similar chelating activity to known phytosiderophores []. Further research is needed to fully understand the relationship between specific structural modifications and iron-chelating capacity.
Q7: What analytical techniques are used to quantify DMA in plant and soil samples?
A7: High-performance liquid chromatography (HPLC) coupled with various detection methods, such as UV-Vis or mass spectrometry (MS), is widely employed for DMA quantification [, , , ]. The development of a 13C4-labeled DMA internal standard has further enabled accurate quantification using isotope dilution techniques [].
Q8: What is the environmental fate of DMA and its potential impact?
A8: DMA is biodegradable in the soil, and its degradation rate is influenced by factors like microbial activity and soil properties []. Research suggests that DMA might play a role in mitigating cadmium (Cd) uptake in plants [].
Q9: How does the solubility of DMA affect its bioavailability to plants?
A9: DMA is a highly water-soluble compound, enabling its efficient movement through the soil solution and facilitating uptake by plant roots [].
試験管内研究製品の免責事項と情報
BenchChemで提示されるすべての記事および製品情報は、情報提供を目的としています。BenchChemで購入可能な製品は、生体外研究のために特別に設計されています。生体外研究は、ラテン語の "in glass" に由来し、生物体の外で行われる実験を指します。これらの製品は医薬品または薬として分類されておらず、FDAから任何の医療状態、病気、または疾患の予防、治療、または治癒のために承認されていません。これらの製品を人間または動物に体内に導入する形態は、法律により厳格に禁止されています。これらのガイドラインに従うことは、研究と実験において法的および倫理的な基準の遵守を確実にするために重要です。