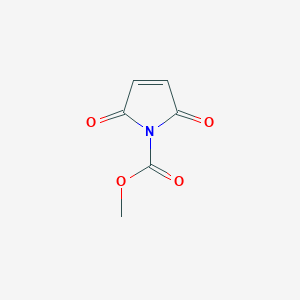
N-Methoxycarbonylmaleimide
概要
説明
Synthesis Analysis
The synthesis of N-Methoxycarbonylmaleimide-related compounds often involves regioselective reduction processes. For example, 3-Methoxymaleimide and its N-alkyl derivatives can be synthesized by base-promoted N-alkylation, followed by reduction with sodium borohydride to yield methyl 5-hydroxytetramates, which are useful intermediates in synthesizing a variety of tetramate derivatives (Issa et al., 2006).
Molecular Structure Analysis
The molecular structure of N-Methoxycarbonylmaleimide derivatives has been elucidated through various structural studies. For instance, cycloadducts formed with maleic anhydride and N-methylmaleimide show the degree of C-C bond lengthening, which correlates with their reactivity towards retro-Diels-Alder reactions (Goh et al., 2008).
Chemical Reactions and Properties
N-Methoxycarbonylmaleimide undergoes various chemical reactions, including spontaneous alternating copolymerization with methoxyallene to produce alternating copolymers and adducts, showcasing its reactivity and versatility in polymer chemistry (Yamamoto et al., 1999).
科学的研究の応用
N-Hydroxysuccinimide, a related compound, demonstrates a chemically reversible redox response, serving as a mediator for the oxidation of benzyl alcohol in specific conditions (Kishioka & Yamada, 2006).
N-Phenylmaleimide, another derivative, has been studied for its reaction with methanol, leading to the production of specific compounds with potential applications in organic chemistry (Finnegan & Mueller, 1965).
Certain derivatives of N-phenylmaleimide have shown low toxicity and the ability to inhibit melanoma growth, suggesting potential as leads for developing new anticancer agents (Noldin et al., 2015).
N-Cyclohexylmaleimide has been synthesized for applications in heat-resistant transparent methacrylic resin (Kita, Kishino, & Nakagawa, 1997).
The Diels-Alder reactions involving N-phenylmaleimide and 2(1H)-pyridones under specific conditions are studied for the production of isoquinuclidine derivatives (Hoshino, Matsuzaki, & Fujita, 2008).
Maleimido acids and maleoylpeptides are used for preparing a wide range of biochemically interesting conjugates due to their reactivity toward thiol groups, indicating potential applications in biochemistry and molecular biology (Keller & Rudinger, 1975).
The study of copolymerization of N-carbethoxymaleimide with divinyl ether indicates applications in polymer science, particularly in the creation of polymers with diverse molecular weights and comonomer ratios (Butler & Zampini, 1977).
A study on the reaction of thioacetamide with N-arylmaleimides producing various compounds suggests applications in organic synthesis and pharmaceutical chemistry (Aseeva et al., 2022).
The regioselective synthesis of methyl 5-hydroxytetramates from 3-methoxymaleimides offers potential applications in the synthesis of various tetramate derivatives (Issa et al., 2006).
Enzyme-activated surfactants for the dispersion of carbon nanotubes represent an application in materials science and nanotechnology (Cousins et al., 2009).
Radical polymerization studies of n-(ethoxycarbonylphenyl)maleimides indicate applications in polymer chemistry, particularly in creating polymers with excellent thermal stability and solubility in various solvents (Matsumoto, Oki, & Otsu, 1992).
Vinyl chloride/N-phenylmaleimide copolymerization studies highlight applications in improving heat resistance and homogeneity in PVC, relevant in materials science and polymer technology (Du et al., 2000).
Reactions of methoxy-(2-trimethylsilyl)ethoxycarbene with various functional groups, including Michael acceptors, are significant in organic chemistry and synthesis (Pole, Sharma, & Warkentin, 1996).
Supramolecular hydrogels utilizing derivatives of N-methoxycarbonylmaleimide have applications in ligand-receptor interaction studies, thermal and pH perturbation, and chiral recognition, indicating potential in biochemical and pharmaceutical research (Zhang et al., 2003).
A study on a methotrexate binding protein fraction from L1210 lymphocyte plasma membranes, using derivatives of N-methoxycarbonylmaleimide, suggests potential applications in cancer research and therapy (McCormick et al., 1979).
Bismaleimides treated with BDA yield novel bis-type 7-azanorbornane derivatives, indicating applications in organic synthesis and pharmaceuticals (Zaima, Matsuno, & Mitsuhashi, 1988).
Preparation of maleimide-modified oligonucleotides using N-Methoxycarbonylmaleimide provides a method with high control over the final construct, relevant in molecular biology and genetic engineering (Kjærsgaard, Hansen, & Gothelf, 2022).
A mitochondria-immobilized fluorescent probe developed using derivatives of N-methoxycarbonylmaleimide effectively detects hypochlorite in living cells, tissues, and zebrafish, showing potential in biological imaging and diagnostics (Li et al., 2020).
Safety and Hazards
When handling N-Methoxycarbonylmaleimide, it is advised to avoid dust formation, breathing mist, gas or vapours, and contact with skin and eyes. Use of personal protective equipment and chemical impermeable gloves is recommended. Ensure adequate ventilation, remove all sources of ignition, and evacuate personnel to safe areas .
将来の方向性
作用機序
Target of Action
The primary target of N-Methoxycarbonylmaleimide is proteins, specifically those involved in protein-protein interactions . The compound binds to these proteins, affecting their function and interaction with other proteins .
Mode of Action
N-Methoxycarbonylmaleimide acts as a reversible inhibitor of protein-protein interactions . It binds to proteins in a non-covalent manner, mediated by hydrogen bonds and hydrophobic interactions . This binding can alter the function of the proteins and disrupt their interactions with other proteins .
Biochemical Pathways
N-Methoxycarbonylmaleimide affects the biosynthesis of chitin and β(1,3)glucan, components of the fungal cell wall . The membrane enzyme, β(1,3)glucan synthase, has been proposed as a putative primary target of N-substituted maleimides in Candida albicans cells . This suggests that N-Methoxycarbonylmaleimide may have antifungal properties by disrupting the synthesis of key components of the fungal cell wall .
Pharmacokinetics
The compound’s molecular weight (15511 g/mol ) and its solid form at room temperature suggest that it may have good bioavailability .
Result of Action
The molecular and cellular effects of N-Methoxycarbonylmaleimide’s action are largely dependent on the specific proteins it targets. By inhibiting protein-protein interactions, it can disrupt various cellular processes, potentially leading to cell death . In the context of fungi, the inhibition of β(1,3)glucan synthase can lead to a weakened cell wall and increased susceptibility to antifungal agents .
Action Environment
The action, efficacy, and stability of N-Methoxycarbonylmaleimide can be influenced by various environmental factors. For instance, the pH and temperature of the environment can affect the compound’s stability and its ability to bind to proteins . Additionally, the presence of other molecules can either compete with or facilitate the compound’s binding to its target proteins.
特性
IUPAC Name |
methyl 2,5-dioxopyrrole-1-carboxylate | |
---|---|---|
Source | PubChem | |
URL | https://pubchem.ncbi.nlm.nih.gov | |
Description | Data deposited in or computed by PubChem | |
InChI |
InChI=1S/C6H5NO4/c1-11-6(10)7-4(8)2-3-5(7)9/h2-3H,1H3 | |
Source | PubChem | |
URL | https://pubchem.ncbi.nlm.nih.gov | |
Description | Data deposited in or computed by PubChem | |
InChI Key |
LLAZQXZGAVBLRX-UHFFFAOYSA-N | |
Source | PubChem | |
URL | https://pubchem.ncbi.nlm.nih.gov | |
Description | Data deposited in or computed by PubChem | |
Canonical SMILES |
COC(=O)N1C(=O)C=CC1=O | |
Source | PubChem | |
URL | https://pubchem.ncbi.nlm.nih.gov | |
Description | Data deposited in or computed by PubChem | |
Molecular Formula |
C6H5NO4 | |
Source | PubChem | |
URL | https://pubchem.ncbi.nlm.nih.gov | |
Description | Data deposited in or computed by PubChem | |
DSSTOX Substance ID |
DTXSID70204311 | |
Record name | Methyl 2,5-dihydro-2,5-dioxo-1H-pyrrole-1-carboxylate | |
Source | EPA DSSTox | |
URL | https://comptox.epa.gov/dashboard/DTXSID70204311 | |
Description | DSSTox provides a high quality public chemistry resource for supporting improved predictive toxicology. | |
Molecular Weight |
155.11 g/mol | |
Source | PubChem | |
URL | https://pubchem.ncbi.nlm.nih.gov | |
Description | Data deposited in or computed by PubChem | |
CAS RN |
55750-48-6 | |
Record name | N-(Methoxycarbonyl)maleimide | |
Source | CAS Common Chemistry | |
URL | https://commonchemistry.cas.org/detail?cas_rn=55750-48-6 | |
Description | CAS Common Chemistry is an open community resource for accessing chemical information. Nearly 500,000 chemical substances from CAS REGISTRY cover areas of community interest, including common and frequently regulated chemicals, and those relevant to high school and undergraduate chemistry classes. This chemical information, curated by our expert scientists, is provided in alignment with our mission as a division of the American Chemical Society. | |
Explanation | The data from CAS Common Chemistry is provided under a CC-BY-NC 4.0 license, unless otherwise stated. | |
Record name | Methyl 2,5-dihydro-2,5-dioxo-1H-pyrrole-1-carboxylate | |
Source | ChemIDplus | |
URL | https://pubchem.ncbi.nlm.nih.gov/substance/?source=chemidplus&sourceid=0055750486 | |
Description | ChemIDplus is a free, web search system that provides access to the structure and nomenclature authority files used for the identification of chemical substances cited in National Library of Medicine (NLM) databases, including the TOXNET system. | |
Record name | Methyl 2,5-dihydro-2,5-dioxo-1H-pyrrole-1-carboxylate | |
Source | EPA DSSTox | |
URL | https://comptox.epa.gov/dashboard/DTXSID70204311 | |
Description | DSSTox provides a high quality public chemistry resource for supporting improved predictive toxicology. | |
Record name | Methyl 2,5-dihydro-2,5-dioxo-1H-pyrrole-1-carboxylate | |
Source | European Chemicals Agency (ECHA) | |
URL | https://echa.europa.eu/substance-information/-/substanceinfo/100.054.339 | |
Description | The European Chemicals Agency (ECHA) is an agency of the European Union which is the driving force among regulatory authorities in implementing the EU's groundbreaking chemicals legislation for the benefit of human health and the environment as well as for innovation and competitiveness. | |
Explanation | Use of the information, documents and data from the ECHA website is subject to the terms and conditions of this Legal Notice, and subject to other binding limitations provided for under applicable law, the information, documents and data made available on the ECHA website may be reproduced, distributed and/or used, totally or in part, for non-commercial purposes provided that ECHA is acknowledged as the source: "Source: European Chemicals Agency, http://echa.europa.eu/". Such acknowledgement must be included in each copy of the material. ECHA permits and encourages organisations and individuals to create links to the ECHA website under the following cumulative conditions: Links can only be made to webpages that provide a link to the Legal Notice page. | |
Synthesis routes and methods I
Procedure details
Synthesis routes and methods II
Procedure details
Synthesis routes and methods III
Procedure details
Retrosynthesis Analysis
AI-Powered Synthesis Planning: Our tool employs the Template_relevance Pistachio, Template_relevance Bkms_metabolic, Template_relevance Pistachio_ringbreaker, Template_relevance Reaxys, Template_relevance Reaxys_biocatalysis model, leveraging a vast database of chemical reactions to predict feasible synthetic routes.
One-Step Synthesis Focus: Specifically designed for one-step synthesis, it provides concise and direct routes for your target compounds, streamlining the synthesis process.
Accurate Predictions: Utilizing the extensive PISTACHIO, BKMS_METABOLIC, PISTACHIO_RINGBREAKER, REAXYS, REAXYS_BIOCATALYSIS database, our tool offers high-accuracy predictions, reflecting the latest in chemical research and data.
Strategy Settings
Precursor scoring | Relevance Heuristic |
---|---|
Min. plausibility | 0.01 |
Model | Template_relevance |
Template Set | Pistachio/Bkms_metabolic/Pistachio_ringbreaker/Reaxys/Reaxys_biocatalysis |
Top-N result to add to graph | 6 |
Feasible Synthetic Routes
Q & A
Q1: What is the primary application of N-Methoxycarbonylmaleimide in bioconjugation chemistry?
A1: N-Methoxycarbonylmaleimide is a valuable reagent for conjugating molecules, particularly in oligonucleotide modification. [, ] It reacts efficiently with amine groups, allowing for the direct introduction of a maleimide functionality onto a molecule. This maleimide group can then react with thiol groups, enabling the conjugation of the molecule to other thiol-containing molecules, such as proteins. []
Q2: How does the reactivity of N-Methoxycarbonylmaleimide compare to traditional heterobifunctional linkers used for similar conjugation reactions?
A2: Studies have shown that oligonucleotides modified with N-Methoxycarbonylmaleimide exhibit comparable reactivity to those modified with the traditional heterobifunctional linker, 4-(N-maleimidomethyl)-cyclohexane-1-carboxylate (SMCC), when conjugated to bovine serum albumin. [] This suggests that N-Methoxycarbonylmaleimide provides a viable alternative to conventional methods, offering a simpler approach without compromising reactivity.
Q3: Can you provide an example of a successful application of N-Methoxycarbonylmaleimide in radiolabeling?
A3: Researchers successfully synthesized a novel fluorine-18 labeled reagent, 1-[3-(2-[18F]fluoropyridin-3-yloxy)propyl]pyrrole-2,5-dione ([(18)F]FPyME), using N-Methoxycarbonylmaleimide. [] This reagent utilizes the reactivity of the maleimide group for chemoselective conjugation with thiol-containing peptides and proteins. The incorporation of fluorine-18 allows for its application in Positron Emission Tomography (PET) imaging.
Q4: What are the advantages of using N-Methoxycarbonylmaleimide in the synthesis of [(18)F]FPyME compared to other radiolabeling strategies?
A4: [(18)F]FPyME demonstrates high chemoselectivity for thiol groups over amine groups, offering a significant advantage over less selective [(18)F] reagents that target both functionalities. [] This selectivity is crucial when working with complex biomolecules like proteins, ensuring the radiolabel is introduced at the desired location without unwanted side reactions. This precise labeling enables the development of more targeted and effective radiopharmaceuticals for PET imaging.
試験管内研究製品の免責事項と情報
BenchChemで提示されるすべての記事および製品情報は、情報提供を目的としています。BenchChemで購入可能な製品は、生体外研究のために特別に設計されています。生体外研究は、ラテン語の "in glass" に由来し、生物体の外で行われる実験を指します。これらの製品は医薬品または薬として分類されておらず、FDAから任何の医療状態、病気、または疾患の予防、治療、または治癒のために承認されていません。これらの製品を人間または動物に体内に導入する形態は、法律により厳格に禁止されています。これらのガイドラインに従うことは、研究と実験において法的および倫理的な基準の遵守を確実にするために重要です。