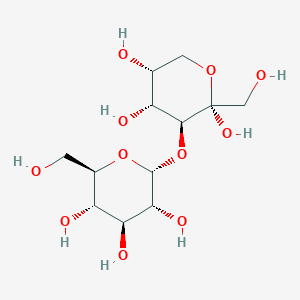
D-(+)-Turanose
- 専門家チームからの見積もりを受け取るには、QUICK INQUIRYをクリックしてください。
- 品質商品を競争力のある価格で提供し、研究に集中できます。
説明
科学的研究の応用
Turanose has a wide range of scientific research applications. In chemistry, it is used as a functional sweetener or bulking agent in food processing due to its mild taste, low calorie content, and anti-cariogenic properties . In biology, turanose is involved in intracellular carbohydrate signaling and can be used as a carbon source by various organisms, including bacteria and fungi . In industry, turanose is used in the detection of pathogens, facilitation of cellular respiration, and authentication of honey .
作用機序
ツラノースは、細胞内炭水化物シグナル伝達への関与を通じてその効果を発揮します。 高等植物では、スクローストランスポーターの作用によって獲得され、さまざまな生物の炭素源として使用できます . シグナル伝達の役割に加えて、ツラノースは細胞呼吸を促進し、炎症を調節します . これらのプロセスに関与する分子標的と経路には、スクローストランスポーターと、炭水化物代謝に関連するさまざまなシグナル伝達経路が含まれます .
準備方法
ツラノースは、化学的および生物学的手段の両方で生成することができます。 一般的な方法の1つは、スクロースからツラノースの合成を触媒するアミロスクラーゼ酵素を使用することです . 反応条件は通常、スクロースの水溶液を含み、アミロスクラーゼで処理して高純度のツラノースを生成します . 工業生産方法では、ツラノースの効率的かつスケーラブルな生産を確保するために、酵素プロセスも使用されています .
化学反応の分析
ツラノースは、加水分解、酸化、還元など、さまざまな化学反応を受けます。 消化管では、ツラノースは比較的安定しており、有意に加水分解されません . 人工的な小腸環境では、ツラノースは4時間後にスクロースが36%加水分解されると、約18%分解されます . ツラノースは、非酵素的褐変反応や動的蒸気吸着にも関与します . これらの反応で使用される一般的な試薬および条件には、酸性または塩基性環境、および高温が含まれます . これらの反応から生成される主要な生成物は、使用される特定の条件と試薬によって異なります。
科学研究への応用
ツラノースは、幅広い科学研究への応用があります。 化学では、その穏やかな味、低カロリー含有量、抗う蝕性のために、食品加工において機能性甘味料または増量剤として使用されます . 生物学では、ツラノースは細胞内炭水化物シグナル伝達に関与し、細菌や真菌などのさまざまな生物の炭素源として使用できます . 産業では、ツラノースは病原体の検出、細胞呼吸の促進、蜂蜜の認証に使用されます .
類似化合物との比較
特性
{ "Design of the Synthesis Pathway": "The synthesis of D-(+)-Turanose can be achieved through a four-step process involving protection, reduction, acetylation, and deprotection reactions.", "Starting Materials": ["D-glucose", "triethyl orthoformate", "acetic anhydride", "sodium borohydride", "acetic acid", "hydrochloric acid", "sodium hydroxide"], "Reaction": [ "Step 1: Protection of D-glucose using triethyl orthoformate and acetic anhydride to form a triethyl orthoformate derivative", "Step 2: Reduction of the triethyl orthoformate derivative using sodium borohydride in acetic acid to form a protected D-glucose derivative", "Step 3: Acetylation of the protected D-glucose derivative using acetic anhydride and pyridine to form a protected D-(+)-Turanose derivative", "Step 4: Deprotection of the protected D-(+)-Turanose derivative using hydrochloric acid and sodium hydroxide to obtain D-(+)-Turanose" ] } | |
CAS番号 |
547-25-1 |
分子式 |
C12H22O11 |
分子量 |
342.30 g/mol |
IUPAC名 |
1,4,5,6-tetrahydroxy-3-[3,4,5-trihydroxy-6-(hydroxymethyl)oxan-2-yl]oxyhexan-2-one |
InChI |
InChI=1S/C12H22O11/c13-1-4(16)7(18)11(5(17)2-14)23-12-10(21)9(20)8(19)6(3-15)22-12/h4,6-16,18-21H,1-3H2 |
InChIキー |
RULSWEULPANCDV-UHFFFAOYSA-N |
異性体SMILES |
C([C@@H]1[C@H]([C@@H]([C@H]([C@H](O1)O[C@@H]([C@@H]([C@@H](CO)O)O)C(=O)CO)O)O)O)O |
SMILES |
C1C(C(C(C(O1)(CO)O)OC2C(C(C(C(O2)CO)O)O)O)O)O |
正規SMILES |
C(C1C(C(C(C(O1)OC(C(C(CO)O)O)C(=O)CO)O)O)O)O |
melting_point |
168 °C |
547-25-1 | |
物理的記述 |
Solid |
溶解性 |
1000 mg/mL at 25 °C |
同義語 |
3-O-alpha-D-glucopyranosyl-D-fructose turanose turanose, d- |
製品の起源 |
United States |
Q1: Does D-(+)-Turanose exhibit any notable interactions with biological systems?
A1: Yes, research suggests this compound plays a role in the growth and metabolism of various organisms. For instance, it serves as a carbon source for certain bacteria like Lactobacillus salivarus and Lactobacillus fructivorans found in fermenting bananas. [] Additionally, it influences the virulence and morphology of the fungal pathogen Candida albicans, impacting the expression of genes involved in filamentation and the secretion of cell wall components. []
Q2: Are there any studies investigating the effect of this compound on bone health?
A2: Research indicates that this compound might be involved in bone metabolism. A study using the human osteoblast cell line hFOB 1.19 revealed that the cells utilized this compound for growth under both normoxic and hypoxic conditions. This suggests a potential role for this compound in bone cell energy production and metabolism, especially in low-oxygen environments often associated with bone fracture healing. []
Q3: What is the molecular formula and weight of this compound?
A3: The molecular formula of this compound is C12H22O11, and its molecular weight is 342.30 g/mol.
Q4: Is there any spectroscopic data available for this compound?
A4: Yes, 13C Nuclear Magnetic Resonance (NMR) spectroscopy has been utilized to study the anomeric equilibrium of this compound in water. [] This technique provides valuable information about the structure and conformation of the molecule in solution.
試験管内研究製品の免責事項と情報
BenchChemで提示されるすべての記事および製品情報は、情報提供を目的としています。BenchChemで購入可能な製品は、生体外研究のために特別に設計されています。生体外研究は、ラテン語の "in glass" に由来し、生物体の外で行われる実験を指します。これらの製品は医薬品または薬として分類されておらず、FDAから任何の医療状態、病気、または疾患の予防、治療、または治癒のために承認されていません。これらの製品を人間または動物に体内に導入する形態は、法律により厳格に禁止されています。これらのガイドラインに従うことは、研究と実験において法的および倫理的な基準の遵守を確実にするために重要です。