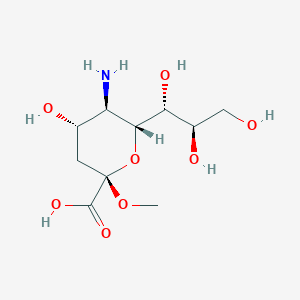
Methyl 5-amino-3,5-dideoxy-D-glycero-beta-D-galacto-2-nonulopyranosidonic acid
説明
Methyl 5-amino-3,5-dideoxy-D-glycero-β-D-galacto-2-nonulopyranosidonic acid is a sialic acid derivative, a class of nine-carbon α-keto acid sugars prominent in glycoproteins and glycolipids. Sialic acids are critical in biological recognition processes, including pathogen-host interactions and immune modulation . This compound is distinguished by its 5-amino group at the C5 position, contrasting with common sialic acids like N-acetylneuraminic acid (Neu5Ac) or N-glycolylneuraminic acid (Neu5Gc), which feature N-acyl modifications (e.g., acetyl or glycolyl) at C5 . The absence of hydroxyl groups at C3 and C5 (due to deoxygenation) further differentiates its structural and conformational properties, impacting its chemical reactivity and biological interactions.
特性
IUPAC Name |
(2S,4S,5R,6R)-5-amino-4-hydroxy-2-methoxy-6-[(1R,2R)-1,2,3-trihydroxypropyl]oxane-2-carboxylic acid | |
---|---|---|
Source | PubChem | |
URL | https://pubchem.ncbi.nlm.nih.gov | |
Description | Data deposited in or computed by PubChem | |
InChI |
InChI=1S/C10H19NO8/c1-18-10(9(16)17)2-4(13)6(11)8(19-10)7(15)5(14)3-12/h4-8,12-15H,2-3,11H2,1H3,(H,16,17)/t4-,5+,6+,7+,8+,10-/m0/s1 | |
Source | PubChem | |
URL | https://pubchem.ncbi.nlm.nih.gov | |
Description | Data deposited in or computed by PubChem | |
InChI Key |
OMVQZDUAKZZNEF-APDUKXCWSA-N | |
Source | PubChem | |
URL | https://pubchem.ncbi.nlm.nih.gov | |
Description | Data deposited in or computed by PubChem | |
Canonical SMILES |
COC1(CC(C(C(O1)C(C(CO)O)O)N)O)C(=O)O | |
Source | PubChem | |
URL | https://pubchem.ncbi.nlm.nih.gov | |
Description | Data deposited in or computed by PubChem | |
Isomeric SMILES |
CO[C@]1(C[C@@H]([C@H]([C@@H](O1)[C@@H]([C@@H](CO)O)O)N)O)C(=O)O | |
Source | PubChem | |
URL | https://pubchem.ncbi.nlm.nih.gov | |
Description | Data deposited in or computed by PubChem | |
Molecular Formula |
C10H19NO8 | |
Source | PubChem | |
URL | https://pubchem.ncbi.nlm.nih.gov | |
Description | Data deposited in or computed by PubChem | |
DSSTOX Substance ID |
DTXSID501309363 | |
Record name | 2-O-Methyl-β-neuraminic acid | |
Source | EPA DSSTox | |
URL | https://comptox.epa.gov/dashboard/DTXSID501309363 | |
Description | DSSTox provides a high quality public chemistry resource for supporting improved predictive toxicology. | |
Molecular Weight |
281.26 g/mol | |
Source | PubChem | |
URL | https://pubchem.ncbi.nlm.nih.gov | |
Description | Data deposited in or computed by PubChem | |
CAS No. |
56144-08-2 | |
Record name | 2-O-Methyl-β-neuraminic acid | |
Source | CAS Common Chemistry | |
URL | https://commonchemistry.cas.org/detail?cas_rn=56144-08-2 | |
Description | CAS Common Chemistry is an open community resource for accessing chemical information. Nearly 500,000 chemical substances from CAS REGISTRY cover areas of community interest, including common and frequently regulated chemicals, and those relevant to high school and undergraduate chemistry classes. This chemical information, curated by our expert scientists, is provided in alignment with our mission as a division of the American Chemical Society. | |
Explanation | The data from CAS Common Chemistry is provided under a CC-BY-NC 4.0 license, unless otherwise stated. | |
Record name | 2-O-Methyl-β-neuraminic acid | |
Source | EPA DSSTox | |
URL | https://comptox.epa.gov/dashboard/DTXSID501309363 | |
Description | DSSTox provides a high quality public chemistry resource for supporting improved predictive toxicology. | |
Record name | Methyl 5-amino-3,5-dideoxy-D-glycero-β-D-galacto-2-nonulopyranosidonic acid | |
Source | European Chemicals Agency (ECHA) | |
URL | https://echa.europa.eu/substance-information/-/substanceinfo/100.054.539 | |
Description | The European Chemicals Agency (ECHA) is an agency of the European Union which is the driving force among regulatory authorities in implementing the EU's groundbreaking chemicals legislation for the benefit of human health and the environment as well as for innovation and competitiveness. | |
Explanation | Use of the information, documents and data from the ECHA website is subject to the terms and conditions of this Legal Notice, and subject to other binding limitations provided for under applicable law, the information, documents and data made available on the ECHA website may be reproduced, distributed and/or used, totally or in part, for non-commercial purposes provided that ECHA is acknowledged as the source: "Source: European Chemicals Agency, http://echa.europa.eu/". Such acknowledgement must be included in each copy of the material. ECHA permits and encourages organisations and individuals to create links to the ECHA website under the following cumulative conditions: Links can only be made to webpages that provide a link to the Legal Notice page. | |
作用機序
Target of Action
Methyl beta-Neuraminic Acid, also known as (2S,4S,5R,6R)-5-AMINO-4-HYDROXY-2-METHOXY-6-[(1R,2R)-1,2,3-TRIHYDROXYPROPYL]OXANE-2-CARBOXYLIC ACID or Methyl 5-amino-3,5-dideoxy-D-glycero-beta-D-galacto-2-nonulopyranosidonic acid, is a derivative of neuraminic acid. Its primary targets include various enzymes such as Tetanus toxin, Sialidase, Endo-N-acetylneuraminidase, and Hemagglutinin-neuraminidase. These enzymes play crucial roles in various biological processes, including viral replication and bacterial infection.
Mode of Action
The compound’s mode of action involves blocking the function of the viral neuraminidase protein (EC 3.2.1.18), thus preventing the virus from budding from the host cell. This interaction with its targets leads to a halt in the life cycle of the virus, thereby inhibiting its spread.
Biochemical Pathways
Methyl beta-Neuraminic Acid affects the biochemical pathways related to the synthesis and function of sialic acids. Sialic acids are a family of monosaccharides commonly presented on the termini of glycans in higher animals and some microorganisms. They play a crucial role in various biological functions, including cell-cell communication, immunity, and pathogenesis.
Result of Action
The result of Methyl beta-Neuraminic Acid’s action is the inhibition of viral replication and spread. By blocking the function of the viral neuraminidase protein, the compound prevents the virus from budding from the host cell, thereby halting the viral life cycle.
Action Environment
The action, efficacy, and stability of Methyl beta-Neuraminic Acid can be influenced by various environmental factors. For instance, the compound’s stability and efficacy might be affected by factors such as temperature, pH, and the presence of other compounds. .
生化学分析
Biochemical Properties
Methyl beta-Neuraminic Acid is involved in several biochemical reactions, primarily as a component of glycoproteins and glycolipids. It interacts with various enzymes, such as sialyltransferases, which catalyze the transfer of sialic acid residues to glycoproteins and glycolipids. This interaction is essential for the formation of sialylated glycoconjugates, which are critical for cell-cell communication and immune response. Additionally, Methyl beta-Neuraminic Acid interacts with neuraminidases, enzymes that cleave sialic acid residues from glycoconjugates, playing a role in the regulation of these molecules .
Cellular Effects
Methyl beta-Neuraminic Acid influences various cellular processes, including cell signaling, gene expression, and cellular metabolism. It is a key component of the glycocalyx, the outer layer of the cell membrane, where it modulates cell-cell interactions and immune recognition. The presence of Methyl beta-Neuraminic Acid on the cell surface can affect cell signaling pathways by interacting with sialic acid-binding immunoglobulin-like lectins (SIGLECs), which are involved in immune regulation . Additionally, it can influence gene expression by modulating the activity of transcription factors and other regulatory proteins.
Molecular Mechanism
At the molecular level, Methyl beta-Neuraminic Acid exerts its effects through various binding interactions with biomolecules. It can bind to specific receptors on the cell surface, such as SIGLECs, modulating immune responses and cell signaling pathways. Furthermore, Methyl beta-Neuraminic Acid can act as a substrate for sialyltransferases and neuraminidases, influencing the synthesis and degradation of sialylated glycoconjugates . These interactions can lead to changes in gene expression and cellular function, contributing to the regulation of various biological processes.
Temporal Effects in Laboratory Settings
In laboratory settings, the effects of Methyl beta-Neuraminic Acid can change over time due to its stability and degradation. Studies have shown that this compound is relatively stable under physiological conditions, but it can be degraded by neuraminidases, leading to the release of free sialic acid . Long-term exposure to Methyl beta-Neuraminic Acid in in vitro and in vivo studies has demonstrated its impact on cellular function, including alterations in cell signaling and immune responses.
Dosage Effects in Animal Models
The effects of Methyl beta-Neuraminic Acid vary with different dosages in animal models. At low doses, it can enhance immune responses and improve cell signaling. At high doses, it may exhibit toxic effects, such as inducing inflammation and disrupting cellular metabolism . Threshold effects have been observed, where a specific dosage range is required to achieve the desired biological effects without causing adverse reactions.
Metabolic Pathways
Methyl beta-Neuraminic Acid is involved in the sialic acid biosynthesis pathway, where it is synthesized from N-acetylmannosamine and phosphoenolpyruvate through a series of enzymatic reactions . It can also be incorporated into glycoproteins and glycolipids through the action of sialyltransferases. The metabolic flux of Methyl beta-Neuraminic Acid can influence the levels of sialylated glycoconjugates, affecting various biological processes.
Transport and Distribution
Within cells and tissues, Methyl beta-Neuraminic Acid is transported and distributed through specific transporters and binding proteins. It can be transported across cell membranes by sialic acid transporters and incorporated into the glycocalyx . The localization and accumulation of Methyl beta-Neuraminic Acid can influence its biological activity and function.
Subcellular Localization
Methyl beta-Neuraminic Acid is primarily localized in the glycocalyx, the outer layer of the cell membrane, where it plays a crucial role in cell-cell interactions and immune recognition . It can also be found in various cellular compartments, including the Golgi apparatus and endoplasmic reticulum, where it is involved in the synthesis and modification of glycoproteins and glycolipids. Post-translational modifications, such as glycosylation, can direct Methyl beta-Neuraminic Acid to specific cellular compartments, influencing its activity and function.
生物活性
Methyl 5-amino-3,5-dideoxy-D-glycero-beta-D-galacto-2-nonulopyranosidonic acid, often referred to as a derivative of nonulosonic acids, is a compound of significant interest in biochemistry and pharmacology due to its biological activity. This article explores its properties, mechanisms of action, and potential applications based on available research findings.
Chemical Structure and Properties
The compound is characterized by its unique structure, which includes:
- Chemical Formula : C11H19NO9
- Molecular Weight : 293.27 g/mol
- Functional Groups : Amino group, hydroxyl groups, and a nonulosonic acid backbone.
This structure is critical for its interaction with biological systems.
-
Inhibition of Pathogen Adhesion :
- This compound has been shown to inhibit the adhesion of certain pathogens to host cells. This is particularly relevant in the context of bacterial infections where adhesion is a critical step in the infection process.
-
Modulation of Immune Responses :
- The compound may influence immune responses by acting on glycan-binding receptors on immune cells. This interaction can modulate cytokine production and enhance immune responses against infections.
-
Antiviral Properties :
- Preliminary studies suggest that this compound exhibits antiviral activity, potentially by interfering with viral entry into host cells or replication processes.
Table 1: Summary of Biological Activities
Case Study: Antibacterial Activity
In a study published in Journal of Medicinal Chemistry, researchers tested the antibacterial efficacy of this compound against Escherichia coli and Staphylococcus aureus. Results indicated a significant reduction in bacterial load when treated with the compound, suggesting its potential as a therapeutic agent against bacterial infections (source: ).
Case Study: Immune Response Modulation
A recent investigation into the immunomodulatory effects of the compound revealed that it enhances the production of pro-inflammatory cytokines such as IL-6 and TNF-alpha in macrophages exposed to lipopolysaccharides (LPS). This suggests a role in boosting innate immune responses (source: ).
科学的研究の応用
Biological Significance
KDN plays a crucial role in cellular functions and is involved in several biological processes:
- Cell Adhesion and Signaling : Sialic acids, including KDN, are often found at the terminal positions of glycoproteins and glycolipids on cell surfaces. They are essential for cell-cell interactions and signaling pathways .
- Tumorigenesis : The expression of KDN is associated with cancer development. It is believed to modulate immune responses and influence tumor progression by altering cell surface properties .
Table 1: Biological Functions of KDN
Function | Description |
---|---|
Cell Adhesion | Facilitates interactions between cells through glycan recognition. |
Immune Modulation | Alters immune cell responses, potentially aiding tumor evasion mechanisms. |
Development Regulation | Involved in normal vertebrate development processes. |
Synthetic Applications
KDN can be synthesized and modified for various applications in research:
- Glycoconjugate Synthesis : KDN is utilized as a building block for synthesizing glycoconjugates, which are essential for studying glycosylation patterns in biomolecules .
- Inhibitors of Glycosyltransferases : Research has shown that derivatives of KDN can act as inhibitors for specific glycosyltransferases, which are enzymes that add sugar moieties to proteins or lipids. This inhibition can be crucial for studying enzyme mechanisms and developing therapeutic agents .
Table 2: Synthetic Applications of KDN
Application | Description |
---|---|
Glycoconjugate Synthesis | Used as a precursor in the synthesis of complex carbohydrates. |
Enzyme Inhibition | Acts as an inhibitor for specific glycosyltransferases, aiding mechanistic studies. |
Metabolic Studies
KDN's role in metabolism has been extensively studied, particularly concerning its biosynthetic pathways:
- Biosynthesis : KDN is synthesized from common precursors through multiple enzymatic steps. Understanding these pathways can provide insights into metabolic disorders where sialic acid metabolism is disrupted .
- Metabolomics : KDN is detected in various biological samples, making it a target for metabolomic studies aimed at understanding disease states and metabolic profiles .
Table 3: Metabolic Pathways Involving KDN
Pathway | Key Enzymes Involved | Implications |
---|---|---|
Sialic Acid Biosynthesis | CMP-Sia synthetase | Regulation of sialic acid levels in tissues. |
Degradation | Sialidases | Impact on cellular signaling and immune response. |
Therapeutic Potential
Research indicates that KDN may have therapeutic applications:
- Cancer Therapy : By modulating sialic acid expression on tumor cells, KDN derivatives could enhance the efficacy of immunotherapies or targeted therapies against cancers .
- Vaccine Development : The incorporation of KDN into vaccine formulations may improve immune responses by mimicking natural glycan structures found on pathogens .
Table 4: Therapeutic Applications of KDN
Application | Description |
---|---|
Cancer Treatment | Potential to enhance immune response against tumors. |
Vaccine Development | Improves immunogenicity when used as an adjuvant or carrier. |
類似化合物との比較
Comparison with Structurally Similar Compounds
The following table summarizes key structural and functional differences between Methyl 5-amino-3,5-dideoxy-D-glycero-β-D-galacto-2-nonulopyranosidonic acid and analogous sialic acid derivatives:
Structural and Functional Analysis
- C5 Substitution: The 5-amino group in the target compound replaces the N-acetyl or N-glycolyl groups seen in Neu5Ac/Neu5Gc. This substitution eliminates steric bulk and introduces a primary amine, enhancing nucleophilicity for chemical conjugation (e.g., coupling to carriers in vaccine design) . However, the absence of N-acyl groups may reduce binding affinity to sialic acid-binding lectins like Siglecs, which recognize acetylated forms .
- This property is exploitable in designing glycomimetic inhibitors.
- Stereochemical Configuration: The β-D-galacto configuration contrasts with the α-D-galacto configuration of most natural sialic acids (e.g., Neu5Acα2–6Gal linkages in human glycans). This β-anomeric preference may confer resistance to mammalian sialidases, which typically cleave α-linked sialosides .
準備方法
Starting Materials and Initial Functionalization
The synthesis typically begins with derivatives of N-acetylneuraminic acid (Neu5Ac). For example, methyl 5-acetamido-3,5-dideoxy-D-glycero-D-galacto-2-nonulopyranosonate serves as a critical precursor. Deacetylation of this compound under acidic conditions (e.g., Dowex-50(H+) in methanol) generates the free amine, which is subsequently protected or modified. Acetic anhydride treatment facilitates re-acetylation or side-chain functionalization.
Stereochemical Control via Intramolecular Cyclization
Intramolecular glycosylation using dimethyl(methylthio)sulfonium triflate (DMTST) has been employed to form pyranose or furanose rings. For instance, treatment of benzyl [methyl 5-N-(O-benzylglycolyl)-3,5-dideoxy-2-thio-α-D-glycero-D-galacto-2-nonulopyranosid]onate with DMTST yields cyclized products with defined stereochemistry. This step is critical for establishing the beta-D-galacto configuration.
Functional Group Transformations
Deacetylation and Amine Generation
The acetamido group at position 5 is deacetylated using sodium methoxide in methanol, producing the free amine. For example, deacetylation of methyl 5-acetamido-3,5-dideoxy-D-glycero-D-galacto-2-nonulopyranosonate (yield: 93%) yields the intermediate methyl 5-amino-3,5-dideoxy-D-glycero-D-galacto-2-nonulopyranosonate . Subsequent hydrolysis with 0.1 N NaOH converts the methyl ester to the free acid.
Reductive Amination and Lactam Formation
Reductive amination of aldehydes derived from ozonolysis of allyl esters introduces secondary amines. For instance, reductive amination of methyl 5-amino-3,5-dideoxy-D-glycero-D-galacto-2-nonulopyranosonate with sodium cyanoborohydride (NaBH3CN) in phosphate buffer (pH 7.0) yields conjugates with controlled substitution. Unintended lactam formation occurs when primary amines undergo intramolecular cyclization, particularly in ester derivatives.
Purification and Characterization
Chromatographic Separation
Silica gel chromatography with eluents such as chloroform-methanol (9:1 v/v) resolves intermediates. For example, benzyl 5-acetamido-4-O-acetyl-8,9-O-isopropylidene-D-glycero-beta-D-galacto-2-nonulopyranosonate is purified using this method before deprotection.
Spectroscopic Validation
-
NMR : Key proton signals include H-4 (δ 4.83 ppm) and H-7 (δ 5.30 ppm) in α-glycosides.
-
X-ray Crystallography : Confirms the chair conformation (2C5) of the pyranose ring and equatorial glycerol side chain.
Reaction Optimization and Yield Data
Step | Reagents/Conditions | Yield (%) | Reference |
---|---|---|---|
Deacetylation | Dowex-50(H+), MeOH | 85–90 | |
Reductive Amination | NaBH3CN, pH 7.0, 37°C | 53* | |
Lactam Formation | EDC, RT | 95 | |
Ester Hydrolysis | 0.1 N NaOH, RT | 93 |
*Yield reported as moles of Neu5Ac per protein conjugate.
Challenges and Mitigation Strategies
Side Reactions in Ester Derivatives
Ester hydrolysis during reductive amination is mitigated by using DMSO as a co-solvent, stabilizing intermediates.
Stereochemical Drift
Low-temperature conditions (–20°C) during glycosylation minimize epimerization.
Industrial-Scale Synthesis Considerations
Q & A
Q. What are the common synthetic routes for Methyl 5-amino-3,5-dideoxy-D-glycero-beta-D-galacto-2-nonulopyranosidonic acid, and what key intermediates are involved?
Methodological Answer: The compound is synthesized via chemo-enzymatic and chemical methods. Chemo-enzymatic routes employ recombinant enzymes like CMP-sialic acid synthetase to activate sialic acid precursors (e.g., Neu5Ac) into CMP-sialic acid donors, which are then transferred to acceptors using sialyltransferases . Chemical methods involve hydrazinolysis to generate 2,3-didehydro intermediates, followed by N-trifluoroacetylation or azide substitution at the C5 position . Key intermediates include 9-O-acetylated sialic acid and acetylated methyl esters of Kdn (2-keto-3-deoxy-D-glycero-D-galacto-nonulosonic acid) .
Q. How is the structural configuration of this compound confirmed in synthetic studies?
Methodological Answer: Structural elucidation relies on nuclear magnetic resonance (NMR) spectroscopy (1H, 13C, 2D COSY, HSQC) to assign stereochemistry and glycosidic linkages. High-resolution mass spectrometry (HRMS) validates molecular weight, with observed m/z values matching calculated masses (e.g., HRMS neg. mode: 1065.4546 for C45H74N6O21S-H) . X-ray crystallography or computational modeling may supplement stereochemical analysis for crystalline derivatives .
Q. What is the biological relevance of this sialic acid derivative in glycobiology research?
Methodological Answer: Sialic acids, including this derivative, mediate virus-host interactions (e.g., influenza binding) and cell recognition via terminal glycan motifs. Modifications like O-acetylation at C7/C9 or C5 amino groups alter receptor specificity and immune evasion, studied using glycan microarrays or surface plasmon resonance (SPR) .
Q. How does the modification at the C5 position (e.g., amino vs. acetamido) influence the compound's physicochemical properties?
Methodological Answer: The C5 substituent modulates hydrophilicity, hydrogen-bonding capacity, and steric effects. Amino groups enhance solubility and electrostatic interactions, while acetyl or glycolyl groups increase metabolic stability and membrane permeability. These properties are critical for designing probes for glycan-binding assays .
Advanced Research Questions
Q. How can regioselective acetylation be achieved during the synthesis of O-acetylated derivatives?
Methodological Answer: Regioselective acetylation is achieved via enzymatic or chemical strategies. Recombinant sialyltransferases selectively modify hydroxyl groups (e.g., C9-O-acetylation) in sialic acid precursors . Chemical methods employ temporary protecting groups like 2-naphthylmethylene to block C7/C8 hydroxyls, enabling selective acetylation at C9 .
Q. What strategies are employed to introduce azide groups into the sialic acid backbone for click chemistry applications?
Methodological Answer: Azide groups are introduced via nucleophilic substitution at C5. For example, 5-azido-5-deoxy-Kdn2en is synthesized by substituting a leaving group (e.g., triflate) with sodium azide, enabling bioorthogonal conjugation via copper-catalyzed azide-alkyne cycloaddition (CuAAC) .
Q. How to address stereochemical challenges in the synthesis of complex sialic acid analogs?
Methodological Answer: Stereochemical control is achieved using oxazolidinthione protecting groups during glycosylation. These groups stabilize the transition state, ensuring α- or β-anomeric configuration retention. For example, methyl 5-N,4-O-thiocarbonyl derivatives maintain α-configuration during glycosidic bond formation .
Q. What enzymatic tools are critical for constructing sialylated glycoconjugates containing this compound?
Methodological Answer: Recombinant enzymes like CMP-sialic acid synthetases (e.g., from Neisseria meningitidis) activate sialic acid into CMP donors, while α-2,3/6/8-sialyltransferases transfer the donor to galactose or N-acetylgalactosamine acceptors. Enzyme engineering (e.g., substrate promiscuity) expands the range of non-natural acceptors .
Q. What analytical challenges arise in characterizing highly O-acetylated sialic acid derivatives, and how are they resolved?
Methodological Answer: O-Acetyl groups are base-labile and prone to migration. Mild electrospray ionization mass spectrometry (ESI-MS) with ammonium acetate buffers prevents hydrolysis. 2D NMR (e.g., ROESY) resolves labile proton environments, while kinetic studies track acetyl migration under varying pH conditions .
Q. How can chemoenzymatic approaches be optimized for scalable production of sialic acid analogs with unnatural modifications?
Methodological Answer: Optimization involves enzyme engineering (e.g., directed evolution for enhanced donor/acceptor promiscuity) and reaction condition tuning (e.g., pH 7.5–8.5, Mn²⁺ cofactors). For example, α-2,8-sialyltransferases with relaxed specificity enable polysialic acid chain elongation on non-natural acceptors .
Featured Recommendations
Most viewed | ||
---|---|---|
Most popular with customers |
試験管内研究製品の免責事項と情報
BenchChemで提示されるすべての記事および製品情報は、情報提供を目的としています。BenchChemで購入可能な製品は、生体外研究のために特別に設計されています。生体外研究は、ラテン語の "in glass" に由来し、生物体の外で行われる実験を指します。これらの製品は医薬品または薬として分類されておらず、FDAから任何の医療状態、病気、または疾患の予防、治療、または治癒のために承認されていません。これらの製品を人間または動物に体内に導入する形態は、法律により厳格に禁止されています。これらのガイドラインに従うことは、研究と実験において法的および倫理的な基準の遵守を確実にするために重要です。