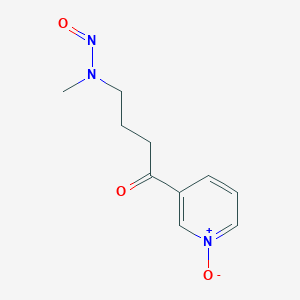
4-(Methylnitrosamino)-1-(3-pyridyl-N-oxide)-1-butanone
概要
説明
4-(Methylnitrosamino)-1-(3-pyridyl-N-oxide)-1-butanone is a tobacco-specific nitrosamine known for its potent carcinogenic properties. It is primarily found in tobacco products and is a significant contributor to the carcinogenicity of tobacco smoke .
準備方法
Synthetic Routes and Reaction Conditions
The synthesis of 4-(Methylnitrosamino)-1-(3-pyridyl-N-oxide)-1-butanone involves the nitrosation of nicotine-derived nitrosamines. The process typically includes the reaction of nicotine with nitrosating agents under acidic conditions .
Industrial Production Methods
Industrial production of this compound is not common due to its carcinogenic nature. it can be synthesized in laboratory settings for research purposes using controlled conditions to ensure safety and minimize exposure .
化学反応の分析
Types of Reactions
4-(Methylnitrosamino)-1-(3-pyridyl-N-oxide)-1-butanone undergoes various chemical reactions, including:
Oxidation: This reaction involves the conversion of the compound into its corresponding N-oxide form.
Reduction: Reduction reactions can convert the nitroso group into an amine group.
Substitution: The compound can undergo nucleophilic substitution reactions, particularly at the pyridyl ring.
Common Reagents and Conditions
Oxidation: Common oxidizing agents include hydrogen peroxide and peracids.
Reduction: Reducing agents such as sodium borohydride or catalytic hydrogenation are used.
Substitution: Nucleophiles like amines or thiols can be used under basic conditions.
Major Products Formed
Oxidation: Formation of N-oxide derivatives.
Reduction: Formation of amine derivatives.
Substitution: Formation of substituted pyridyl derivatives.
科学的研究の応用
Carcinogenic Mechanisms
NNK is recognized for its role in the development of various cancers, particularly lung cancer. It undergoes metabolic activation to form reactive species that can damage DNA, leading to mutations and tumorigenesis.
- Metabolic Activation : NNK is metabolized in the liver to form highly reactive intermediates that can bind to DNA, resulting in mutagenic adducts. This process has been extensively documented in animal models and human studies, highlighting the compound's carcinogenic potential .
- Tumor Promotion : Studies have shown that NNK not only initiates carcinogenesis but also promotes tumor growth through various pathways, including the activation of signaling pathways involved in cell proliferation and survival .
Immunosuppressive Effects
NNK has been shown to induce immunosuppression, which may facilitate tumor development by impairing the body's ability to mount an effective immune response against cancer cells.
- Research Findings : In A/J mice, NNK exposure led to significant suppression of both humoral and cellular immune responses. Nonsteroidal anti-inflammatory drugs (NSAIDs) were found to mitigate these immunosuppressive effects, suggesting a potential therapeutic avenue for counteracting NNK-induced immune dysfunction .
Case Studies and Experimental Research
Numerous studies have investigated the effects of NNK on various biological systems:
- Lung Tumorigenesis : Research has demonstrated that chronic exposure to NNK results in lung tumors in rodent models. For instance, a study indicated that low-dose computed tomography (CT) screening could reduce lung cancer mortality among high-risk populations exposed to NNK .
- Chemoprevention Studies : Investigations into chemopreventive agents have shown promise in reducing NNK-induced tumorigenesis. Agents like N-acetylcysteine have been studied for their protective effects against oxidative stress and DNA damage caused by NNK .
Environmental and Public Health Implications
NNK is not only a concern for smokers but also poses risks through secondhand and thirdhand smoke exposure:
- Thirdhand Smoke Metabolite : As a metabolite of tobacco smoke, NNK can persist on surfaces long after smoking has ceased. This raises concerns about non-smokers' exposure to carcinogenic compounds through contaminated environments .
作用機序
The carcinogenicity of 4-(Methylnitrosamino)-1-(3-pyridyl-N-oxide)-1-butanone is primarily due to its ability to form DNA adducts. The compound undergoes metabolic activation to form reactive intermediates that alkylate DNA, leading to mutations and cancer development. The primary molecular targets include guanine bases in DNA, and the pathways involved are those related to DNA repair and apoptosis .
類似化合物との比較
Similar Compounds
4-(Methylnitrosamino)-1-(3-pyridyl)-1-butanone: Another tobacco-specific nitrosamine with similar carcinogenic properties.
N-Nitrosodimethylamine: A nitrosamine with potent carcinogenic effects, commonly found in various environmental sources.
N-Nitrosopyrrolidine: A nitrosamine known for its carcinogenicity in animal models
Uniqueness
4-(Methylnitrosamino)-1-(3-pyridyl-N-oxide)-1-butanone is unique due to its specific presence in tobacco products and its potent carcinogenicity. Its ability to form DNA adducts and induce lung tumors in animal models makes it a critical compound for studying tobacco-related cancers .
生物活性
4-(Methylnitrosamino)-1-(3-pyridyl-N-oxide)-1-butanone, commonly known as NNK, is a potent tobacco-specific nitrosamine recognized for its carcinogenic properties. This compound is primarily formed during the curing and processing of tobacco and has been extensively studied due to its significant role in tobacco-related cancers, particularly lung cancer. This article explores the biological activity of NNK, including its mechanisms of action, metabolic pathways, and associated toxicological effects.
- Molecular Formula : C10H13N3O3
- Molecular Weight : 223.23 g/mol
- CAS Number : 76014-82-9
Mechanisms of Carcinogenicity
NNK exerts its carcinogenic effects through several mechanisms:
- DNA Adduct Formation : NNK is metabolically activated to form DNA adducts, which can lead to mutations and ultimately cancer. The primary metabolites involved in this process include 4-hydroxy-1-(3-pyridyl)-1-butanone (HPB) and 4-(methylnitrosamino)-1-(3-pyridyl)-1-butanol (NNAL) .
- Oxidative Stress : NNK exposure induces oxidative stress by generating reactive oxygen species (ROS), which can damage cellular components including DNA, proteins, and lipids. This oxidative damage is a significant contributor to its carcinogenic potential .
- Epigenetic Modifications : Studies have shown that NNK alters DNA methylation patterns in lung tissues, affecting gene expression related to tumorigenesis. For instance, differentially methylated genes linked to lung cancer were identified in mice exposed to NNK .
In Vivo Studies
Research has demonstrated that NNK induces tumors in various animal models, particularly in the lungs and nasal cavities of mice. In one study, A/J mice exposed to NNK showed significant immunosuppression alongside increased tumorigenesis . Another study highlighted that intratracheal administration of NNK resulted in altered gene expression associated with lung carcinogenesis .
In Vitro Studies
Cell lines exposed to NNK have shown increased proliferation rates and resistance to apoptosis, further supporting its role as a carcinogen. For example, lung cancer cell lines treated with NNK exhibited upregulation of peroxiredoxin (Prdx) I, a protein associated with oxidative stress response and tumor promotion .
Toxicological Effects on Development
Recent investigations into the developmental toxicity of NNK using zebrafish embryos revealed that exposure leads to lethality and morphological malformations. Metabolomic analyses indicated disruptions in purine and pyrimidine metabolism due to NNK exposure, highlighting its detrimental effects during early development stages .
Study on Lung Cancer Induction
A comprehensive study involving rats demonstrated that chronic exposure to NNK led to the development of lung tumors characterized by specific histopathological changes. The study also reported alterations in signaling pathways associated with cell proliferation and survival .
Dietary Interventions
Research exploring dietary compounds has shown potential protective effects against NNK-induced carcinogenesis. For instance, extracts from Purslane were found to significantly reduce tumor growth and improve tissue integrity in rat models exposed to NNK .
Data Table: Summary of Key Findings
Study | Model | Key Findings |
---|---|---|
Hecht et al., 1998 | Mice | Induction of tumors in nasal cavities and lungs; DNA adduct formation confirmed. |
Wei et al., 2016 | Zebrafish | Lethality and malformations observed; metabolic disruptions noted. |
Kutzer et al., 2021 | Rats | Chronic exposure resulted in lung tumors; epigenetic changes documented. |
Current Study | Cell Lines | Increased proliferation and Prdx I expression; oxidative stress response activated. |
特性
IUPAC Name |
N-methyl-N-[4-(1-oxidopyridin-1-ium-3-yl)-4-oxobutyl]nitrous amide | |
---|---|---|
Source | PubChem | |
URL | https://pubchem.ncbi.nlm.nih.gov | |
Description | Data deposited in or computed by PubChem | |
InChI |
InChI=1S/C10H13N3O3/c1-12(11-15)6-3-5-10(14)9-4-2-7-13(16)8-9/h2,4,7-8H,3,5-6H2,1H3 | |
Source | PubChem | |
URL | https://pubchem.ncbi.nlm.nih.gov | |
Description | Data deposited in or computed by PubChem | |
InChI Key |
KRRWRVSPMYAJPF-UHFFFAOYSA-N | |
Source | PubChem | |
URL | https://pubchem.ncbi.nlm.nih.gov | |
Description | Data deposited in or computed by PubChem | |
Canonical SMILES |
CN(CCCC(=O)C1=C[N+](=CC=C1)[O-])N=O | |
Source | PubChem | |
URL | https://pubchem.ncbi.nlm.nih.gov | |
Description | Data deposited in or computed by PubChem | |
Molecular Formula |
C10H13N3O3 | |
Source | PubChem | |
URL | https://pubchem.ncbi.nlm.nih.gov | |
Description | Data deposited in or computed by PubChem | |
DSSTOX Substance ID |
DTXSID40226924 | |
Record name | 1-Butanone, 4-(methylnitrosoamino)-1-(1-oxido-3-pyridinyl)- | |
Source | EPA DSSTox | |
URL | https://comptox.epa.gov/dashboard/DTXSID40226924 | |
Description | DSSTox provides a high quality public chemistry resource for supporting improved predictive toxicology. | |
Molecular Weight |
223.23 g/mol | |
Source | PubChem | |
URL | https://pubchem.ncbi.nlm.nih.gov | |
Description | Data deposited in or computed by PubChem | |
CAS No. |
76014-82-9 | |
Record name | 4-(Methylnitrosamino)-1-(3-pyridyl-N-oxide)-1-butanone | |
Source | CAS Common Chemistry | |
URL | https://commonchemistry.cas.org/detail?cas_rn=76014-82-9 | |
Description | CAS Common Chemistry is an open community resource for accessing chemical information. Nearly 500,000 chemical substances from CAS REGISTRY cover areas of community interest, including common and frequently regulated chemicals, and those relevant to high school and undergraduate chemistry classes. This chemical information, curated by our expert scientists, is provided in alignment with our mission as a division of the American Chemical Society. | |
Explanation | The data from CAS Common Chemistry is provided under a CC-BY-NC 4.0 license, unless otherwise stated. | |
Record name | 4-(Methylnitrosamino)-1-(3-pyridyl-N-oxide)-1-butanone | |
Source | ChemIDplus | |
URL | https://pubchem.ncbi.nlm.nih.gov/substance/?source=chemidplus&sourceid=0076014829 | |
Description | ChemIDplus is a free, web search system that provides access to the structure and nomenclature authority files used for the identification of chemical substances cited in National Library of Medicine (NLM) databases, including the TOXNET system. | |
Record name | 1-Butanone, 4-(methylnitrosoamino)-1-(1-oxido-3-pyridinyl)- | |
Source | EPA DSSTox | |
URL | https://comptox.epa.gov/dashboard/DTXSID40226924 | |
Description | DSSTox provides a high quality public chemistry resource for supporting improved predictive toxicology. | |
Record name | 4-(METHYLNITROSAMINO)-1-(3-PYRIDYL-N-OXIDE)-1-BUTANONE | |
Source | FDA Global Substance Registration System (GSRS) | |
URL | https://gsrs.ncats.nih.gov/ginas/app/beta/substances/L9H5BL53ZJ | |
Description | The FDA Global Substance Registration System (GSRS) enables the efficient and accurate exchange of information on what substances are in regulated products. Instead of relying on names, which vary across regulatory domains, countries, and regions, the GSRS knowledge base makes it possible for substances to be defined by standardized, scientific descriptions. | |
Explanation | Unless otherwise noted, the contents of the FDA website (www.fda.gov), both text and graphics, are not copyrighted. They are in the public domain and may be republished, reprinted and otherwise used freely by anyone without the need to obtain permission from FDA. Credit to the U.S. Food and Drug Administration as the source is appreciated but not required. | |
Record name | 4-(Methylnitrosamino)-1-(1-oxido-3-pyridinyl)-1-butanone | |
Source | Human Metabolome Database (HMDB) | |
URL | http://www.hmdb.ca/metabolites/HMDB0062375 | |
Description | The Human Metabolome Database (HMDB) is a freely available electronic database containing detailed information about small molecule metabolites found in the human body. | |
Explanation | HMDB is offered to the public as a freely available resource. Use and re-distribution of the data, in whole or in part, for commercial purposes requires explicit permission of the authors and explicit acknowledgment of the source material (HMDB) and the original publication (see the HMDB citing page). We ask that users who download significant portions of the database cite the HMDB paper in any resulting publications. | |
Retrosynthesis Analysis
AI-Powered Synthesis Planning: Our tool employs the Template_relevance Pistachio, Template_relevance Bkms_metabolic, Template_relevance Pistachio_ringbreaker, Template_relevance Reaxys, Template_relevance Reaxys_biocatalysis model, leveraging a vast database of chemical reactions to predict feasible synthetic routes.
One-Step Synthesis Focus: Specifically designed for one-step synthesis, it provides concise and direct routes for your target compounds, streamlining the synthesis process.
Accurate Predictions: Utilizing the extensive PISTACHIO, BKMS_METABOLIC, PISTACHIO_RINGBREAKER, REAXYS, REAXYS_BIOCATALYSIS database, our tool offers high-accuracy predictions, reflecting the latest in chemical research and data.
Strategy Settings
Precursor scoring | Relevance Heuristic |
---|---|
Min. plausibility | 0.01 |
Model | Template_relevance |
Template Set | Pistachio/Bkms_metabolic/Pistachio_ringbreaker/Reaxys/Reaxys_biocatalysis |
Top-N result to add to graph | 6 |
Feasible Synthetic Routes
Q1: How is NNK-N-oxide formed in the body?
A1: NNK-N-oxide is generated through the N-oxidation of NNK, primarily catalyzed by cytochrome P450 enzymes (CYPs) [, , , , ]. This metabolic pathway involves the addition of an oxygen atom to the nitrogen of the pyridine ring in NNK.
Q2: Is NNK-N-oxide considered a detoxification product of NNK?
A2: Yes, NNK-N-oxide is generally considered a detoxification product of NNK. Studies suggest that pyridine N-oxidation, the pathway leading to NNK-N-oxide formation, is a less carcinogenic route compared to the α-hydroxylation pathway of NNK [, , , ].
Q3: Which CYP enzymes are primarily involved in the formation of NNK-N-oxide?
A3: Research suggests that multiple CYP enzymes contribute to NNK-N-oxide formation. Studies using human liver microsomes and CYP-overexpressing cell lines have identified CYPs 2A6, 2C8, 2C19, 2E1, and 3A4 as significant contributors to this pathway [, ].
Q4: Can NNK-N-oxide be converted back to NNK?
A4: Yes, there's evidence suggesting that NNK-N-oxide can be reduced back to NNK by certain bacterial species found in the urinary tract. This finding highlights a potential concern for tobacco users with urinary tract infections, as it could lead to increased exposure to the carcinogenic NNK in the bladder [, ].
Q5: How significant is the N-oxidation pathway in the overall metabolism of NNK?
A5: While considered a detoxification pathway, N-oxidation is not the predominant metabolic route for NNK in humans. Studies analyzing urine samples from smokers revealed that NNAL-N-oxide, the N-oxide form of the major NNK metabolite 4-(methylnitrosamino)-1-(3-pyridyl)-1-butanol (NNAL), constituted less than 20% of total NNAL metabolites [].
Q6: Are there species differences in the formation of NNK-N-oxide?
A6: Yes, significant species variations exist in NNK metabolism, including the N-oxidation pathway. For instance, N-oxidation constitutes a larger proportion of total NNK metabolism in Syrian golden hamsters compared to mice and rats [].
Q7: Do dietary factors influence NNK-N-oxide formation?
A7: Research suggests that certain dietary components can modulate NNK metabolism. For example, phenethyl isothiocyanate (PEITC), found in cruciferous vegetables, has been shown to inhibit the α-hydroxylation pathway of NNK but does not affect NNK-N-oxide levels in urine [, , , , ].
Q8: Can NNK-N-oxide be used as a biomarker for NNK exposure?
A8: While detectable in urine, the relatively low levels of NNK-N-oxide compared to other NNK metabolites, coupled with the possibility of bacterial reduction back to NNK, limit its reliability as a sole biomarker for NNK exposure.
Q9: What are the challenges in analyzing NNK-N-oxide?
A9: Quantifying NNK-N-oxide, particularly at low concentrations, poses analytical challenges due to its structural similarity to other NNK metabolites and the potential for interconversion between NNK and its N-oxide form.
Q10: What analytical techniques are used to study NNK-N-oxide?
A10: High-performance liquid chromatography (HPLC) coupled with radiochemical detection is commonly employed to analyze NNK-N-oxide [, ]. The use of high-resolution mass spectrometry (HRMS) further enhances the sensitivity and specificity of these analyses [, ].
試験管内研究製品の免責事項と情報
BenchChemで提示されるすべての記事および製品情報は、情報提供を目的としています。BenchChemで購入可能な製品は、生体外研究のために特別に設計されています。生体外研究は、ラテン語の "in glass" に由来し、生物体の外で行われる実験を指します。これらの製品は医薬品または薬として分類されておらず、FDAから任何の医療状態、病気、または疾患の予防、治療、または治癒のために承認されていません。これらの製品を人間または動物に体内に導入する形態は、法律により厳格に禁止されています。これらのガイドラインに従うことは、研究と実験において法的および倫理的な基準の遵守を確実にするために重要です。