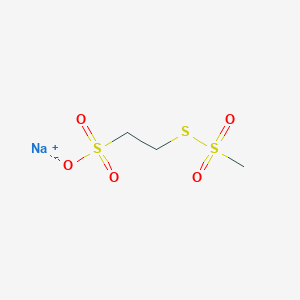
Sodium (2-sulfonatoethyl)methanethiosulfonate
概要
説明
Sodium (2-sulfonatoethyl)methanethiosulfonate (CAS 184644-83-5), often abbreviated as MTSES in literature (though explicitly named here per user requirements), is a sulfhydryl-reactive compound widely utilized in structural and functional studies of membrane proteins. Its structure comprises a methanethiosulfonate group linked to a sulfonatoethyl moiety, conferring a permanent negative charge at physiological pH . This compound reacts specifically and rapidly with cysteine thiol groups to form mixed disulfide bonds, enabling targeted modifications of cysteine-substituted residues in proteins .
MTSES has been instrumental in the substituted cysteine accessibility method (SCAM), a technique for probing solvent accessibility and conformational changes in ion channels, transporters, and receptors. Key applications include:
- GABAA Receptor Studies: MTSES was used to identify solvent-accessible cysteine residues in GABAA receptor channels, revealing critical structural motifs involved in ligand binding and gating .
- Epithelial Na+ Channel (ENaC) Modulation: MTSES altered channel currents in ENaC mutants, highlighting residues critical for ion permeation and regulation .
- Mechanosensitive Channels (MscL): Charged modifications with MTSES increased the sensitivity of MscL channels to membrane tension, demonstrating its utility in engineering stimulus-responsive proteins .
- TMEM43 Channel Characterization: MTSES blocked current in TMEM43 cysteine mutants, confirming the pore-lining role of transmembrane domain 3 .
MTSES is commercially available from suppliers such as Toronto Research Chemicals and Anatrace, with applications validated in diverse systems, including Xenopus oocytes, HEK cells, and bacterial expression models .
準備方法
Synthetic Routes and Reaction Conditions: Sodium (2-sulfonatoethyl)methanethiosulfonate is typically synthesized through the reaction of methanethiosulfonyl chloride with sodium 2-bromoethanesulfonate . The reaction involves the formation of a mixed disulfide linkage, which is then purified to obtain the final product .
Industrial Production Methods: The industrial production of this compound follows similar synthetic routes but on a larger scale. The process involves stringent quality control measures to ensure high purity and consistency of the product .
Types of Reactions:
Substitution Reactions: this compound can participate in nucleophilic substitution reactions, particularly with thiol groups on proteins.
Oxidation and Reduction: The compound can undergo redox reactions, forming mixed disulfide linkages with thiol groups.
Common Reagents and Conditions:
Major Products:
科学的研究の応用
Ion Channel Studies
MTSES has been extensively used in the investigation of ion channel proteins through the method known as "cysteine-scanning mutagenesis." This technique involves substituting specific amino acids with cysteine residues, allowing researchers to study the effects of MTSES on channel activity.
- GABA Receptors : MTSES has been applied to GABAA receptor channels to assess the role of cysteine residues in channel gating and pharmacology. Its application leads to insights into how modifications at these sites can alter receptor function .
- BKCa Channels : Research indicates that MTSES can modulate the activity of large conductance calcium-activated potassium (BKCa) channels. In studies involving guinea-pig myocytes, MTSES was shown to increase the probability of channel opening under certain conditions, providing evidence for its role in channel regulation via S-nitrosylation of cysteine residues .
Protein Folding and Stability
MTSES is also employed in studies related to protein folding and stability. By selectively modifying cysteine residues, researchers can investigate how these modifications affect protein conformation and stability under various physiological conditions.
- Protein Folding Studies : The ability of MTSES to modify specific thiol groups allows for the evaluation of folding pathways and stability across different protein structures. This application is crucial for understanding diseases linked to protein misfolding .
Structural Biology
In structural biology, MTSES serves as a probe for elucidating protein structures through techniques such as X-ray crystallography and nuclear magnetic resonance (NMR) spectroscopy.
- Structural Elucidation : By introducing MTSES at specific sites within proteins, researchers can gain insights into the spatial arrangement of amino acids and how these contribute to overall protein structure and function .
Case Study 1: Modulation of GABA Receptors
A study demonstrated that applying MTSES to GABAA receptors modified the receptor's response to neurotransmitters. This modification provided insights into the allosteric regulation mechanisms of these critical receptors in neurotransmission .
Case Study 2: Investigating BKCa Channel Activity
In another investigation, researchers utilized MTSES to explore its effects on BKCa channel activity in excised membrane patches from guinea-pig taenia caeca. The results indicated that MTSES could enhance channel opening probabilities under specific ionic conditions, highlighting its potential role in modulating cellular excitability .
作用機序
Sodium (2-sulfonatoethyl)methanethiosulfonate exerts its effects by forming mixed disulfide linkages with thiol groups on proteins . This interaction is highly specific to ionized thiolates, making it an effective tool for targeting sulfhydryl groups accessible from the aqueous medium . The compound is particularly useful in studying the structural and functional properties of membrane-associated proteins .
類似化合物との比較
MTSES belongs to the methanethiosulfonate (MTS) reagent family, which includes structurally analogous compounds with varying charges and side chains. Below is a detailed comparison:
Structural and Chemical Properties
Compound Name | Charge | Molecular Weight | Solubility | Reactivity with Thiols |
---|---|---|---|---|
Sodium (2-sulfonatoethyl)methanethiosulfonate (MTSES) | Negative | ~246.2 g/mol | Water-soluble | High (pH-independent) |
[2-(Trimethylammonium)ethyl]methanethiosulfonate bromide (MTSET) | Positive | ~279.2 g/mol | Water-soluble | High (pH-independent) |
(2-Aminoethyl)methanethiosulfonate hydrobromide (MTSEA) | Positive | ~212.1 g/mol | Water-soluble | Moderate (pH-sensitive) |
Benzyl methanethiosulfonate (MTSBn) | Neutral | ~200.3 g/mol | DMSO-soluble | High (hydrophobic environments) |
Key Differences :
- Charge : MTSES is anionic, MTSET and MTSEA are cationic, and MTSBn is neutral. Charge dictates electrostatic interactions with protein environments .
- Size : MTSES and MTSET have bulkier side chains (~8–10 Å) compared to MTSEA (~6 Å), influencing steric accessibility in constrained regions .
- Solubility : Neutral MTSBn requires organic solvents, limiting its use in aqueous systems .
Functional Comparisons in Research Studies
Electrostatic Effects on Reactivity
- Nicotinic Acetylcholine Receptor : Positively charged MTSET reacted faster with binding-site cysteines, while MTSES showed negligible reactivity, consistent with a negatively charged binding pocket .
- ENaC : MTSES reduced currents in αE557C mutants but increased currents in βE499C mutants, whereas MTSET had opposite effects, underscoring residue-specific electrostatic microenvironments .
Size-Dependent Accessibility
- SK Potassium Channels : Smaller MTSEA accessed deeper pore regions compared to bulkier MTSET, challenging the traditional "bundle-crossing" gating model .
生物活性
Sodium (2-sulfonatoethyl)methanethiosulfonate, commonly referred to as MTSES, is a negatively charged, membrane-impermeable methanethiosulfonate (MTS) compound. It has garnered attention in various biological studies due to its unique properties and its role in modulating the activity of ion channels and other proteins through redox modifications.
MTSES functions primarily as a sulfhydryl-reactive agent, modifying cysteine residues in proteins. This modification can affect the conformation and function of various ion channels, particularly large conductance calcium-activated potassium channels (BK channels) and other membrane proteins. The mechanism involves the formation of disulfide bonds or S-nitrosylation, which can lead to alterations in channel activity or protein interactions.
Ion Channel Modulation
- BK Channels : Research has shown that MTSES can significantly increase the probability of opening BK channels under specific conditions. In experiments using excised membrane patches from guinea-pig taenia caeca, MTSES at a concentration of 2.5 mM was found to enhance channel activity, indicating its potential role in influencing smooth muscle contractility through calcium signaling pathways .
- Redox Sensitivity : MTSES has been demonstrated to interact with redox-sensitive sites on ion channels. For instance, the modulation of Cav3.2 T-type calcium channels by MTSES suggests that it can alter channel kinetics through cysteine modification, which is crucial for understanding its role in cellular excitability and signaling .
Case Studies
- Smooth Muscle Function : In a study examining the effects of nitric oxide donors on BK channels, MTSES was used to elucidate the redox mechanisms involved. The results indicated that pre-treatment with MTSES could prevent the excitatory actions of nitric oxide, highlighting its role as a potential therapeutic agent in conditions involving vascular smooth muscle dysfunction .
- Transporter Interactions : Another study explored how MTSES affects the transport activity of amino acid transporters like LAT1 and LAT2. The findings revealed that MTSES could differentially impact substrate transport, suggesting its utility in studying amino acid metabolism and transport mechanisms in various physiological contexts .
Summary of Key Studies
Q & A
Basic Research Questions
Q. What are the primary biochemical applications of Sodium (2-sulfonatoethyl)methanethiosulfonate (MTSES)?
this compound is widely used to modify cysteine residues in proteins via sulfhydryl-specific alkylation. Its negatively charged sulfonate group makes it ideal for studying electrostatic interactions in ion channels, transporters, and redox-sensitive proteins. For example, it has been applied to probe the accessibility of cysteine residues in the CLC-0 chloride channel to assess pore architecture and electrostatic effects on ion selectivity .
Q. How does MTSES differ from other methanethiosulfonate (MTS) reagents in biochemical studies?
MTSES is distinguished by its negative charge, which contrasts with positively charged analogs like MTSET (2-(trimethylammonium)ethyl MTS). This property allows researchers to investigate how electrostatic interactions influence protein function. For instance, in the CLC-0 channel, MTSES modification rates were faster than MTSET, revealing pore regions with preferential anion permeability .
Q. What experimental precautions are necessary when handling MTSES?
Key precautions include:
- Reducing agent removal : Pre-treat protein samples with DTT or TCEP to reduce disulfide bonds and expose free thiols.
- pH control : Perform reactions in slightly alkaline buffers (pH 7.5–8.5) to enhance thiolate reactivity.
- Quenching : Terminate reactions with excess β-mercaptoethanol or cysteine to prevent over-modification .
Advanced Research Questions
Q. How can MTSES be integrated into studies of protein conformational dynamics?
MTSES is used in substituted cysteine accessibility method (SCAM) to map solvent-accessible regions in proteins. By introducing cysteine mutations and monitoring MTSES reactivity via electrophysiology or mass spectrometry, researchers can infer structural rearrangements during gating or ligand binding. For example, in potassium channels, MTSES modification of engineered cysteines revealed movements in the selectivity filter during ion conduction .
Q. How should researchers address contradictory data when using MTSES alongside other MTS reagents?
Contradictions may arise from differences in reagent charge, steric bulk, or reaction kinetics. To resolve these:
- Control experiments : Compare modification rates of MTSES and MTSET under identical conditions.
- Structural modeling : Use computational tools (e.g., MD simulations) to assess steric/electrostatic compatibility of reagents with the target site.
- Functional assays : Validate modifications using complementary techniques like fluorescence quenching or electrophysiology .
Q. What are the limitations of MTSES in studying membrane proteins?
- Membrane permeability : MTSES’s negative charge limits its ability to penetrate lipid bilayers. This restricts its use to extracellular or solvent-accessible cysteine residues.
- Reaction efficiency : Competing endogenous thiols (e.g., glutathione) in cellular systems may reduce specificity.
Solutions include using impermeant derivatives or performing experiments in reconstituted lipid systems .
Q. How can MTSES be applied to study redox-sensitive proteins?
MTSES traps transiently reduced cysteine residues in disulfide-bonded proteins, enabling identification of redox-active sites. For example, in thioredoxin-family proteins, MTSES alkylation followed by proteolysis and MS/MS analysis revealed dynamic disulfide intermediates during catalysis .
Q. Methodological Considerations
Table 1: Key Experimental Parameters for MTSES Applications
Q. Data Interpretation and Validation
Q. How can mass spectrometry validate MTSES-mediated modifications?
- Intact protein analysis : Detect mass shifts (+136 Da per modification) using MALDI-TOF or ESI-MS.
- Peptide mapping : Digest modified proteins with trypsin, then identify alkylated peptides via LC-MS/MS. This confirms site-specific reactivity .
Q. What controls are essential for interpreting electrophysiology data post-MTSES modification?
- Baseline activity : Record currents before MTSES application.
- Reversibility test : Apply reducing agents (e.g., DTT) to confirm disulfide bond formation.
- Non-reactive mutant : Use a cysteine-null mutant to rule out nonspecific effects .
Q. Advanced Structural Insights
Q. How does MTSES inform molecular dynamics (MD) simulations of ion channels?
MD simulations incorporating MTSES-modified cysteine residues can predict electrostatic potential maps and ion occupancy. For example, in K⁺ channels, MTSES modification data aligned with simulations showing anion exclusion from the selectivity filter due to repulsive forces .
特性
IUPAC Name |
sodium;2-methylsulfonylsulfanylethanesulfonate | |
---|---|---|
Source | PubChem | |
URL | https://pubchem.ncbi.nlm.nih.gov | |
Description | Data deposited in or computed by PubChem | |
InChI |
InChI=1S/C3H8O5S3.Na/c1-10(4,5)9-2-3-11(6,7)8;/h2-3H2,1H3,(H,6,7,8);/q;+1/p-1 | |
Source | PubChem | |
URL | https://pubchem.ncbi.nlm.nih.gov | |
Description | Data deposited in or computed by PubChem | |
InChI Key |
NZSYKLNULKPWIX-UHFFFAOYSA-M | |
Source | PubChem | |
URL | https://pubchem.ncbi.nlm.nih.gov | |
Description | Data deposited in or computed by PubChem | |
Canonical SMILES |
CS(=O)(=O)SCCS(=O)(=O)[O-].[Na+] | |
Source | PubChem | |
URL | https://pubchem.ncbi.nlm.nih.gov | |
Description | Data deposited in or computed by PubChem | |
Molecular Formula |
C3H7NaO5S3 | |
Source | PubChem | |
URL | https://pubchem.ncbi.nlm.nih.gov | |
Description | Data deposited in or computed by PubChem | |
DSSTOX Substance ID |
DTXSID00393166 | |
Record name | MTSES | |
Source | EPA DSSTox | |
URL | https://comptox.epa.gov/dashboard/DTXSID00393166 | |
Description | DSSTox provides a high quality public chemistry resource for supporting improved predictive toxicology. | |
Molecular Weight |
242.3 g/mol | |
Source | PubChem | |
URL | https://pubchem.ncbi.nlm.nih.gov | |
Description | Data deposited in or computed by PubChem | |
CAS No. |
184644-83-5 | |
Record name | MTSES | |
Source | EPA DSSTox | |
URL | https://comptox.epa.gov/dashboard/DTXSID00393166 | |
Description | DSSTox provides a high quality public chemistry resource for supporting improved predictive toxicology. | |
Retrosynthesis Analysis
AI-Powered Synthesis Planning: Our tool employs the Template_relevance Pistachio, Template_relevance Bkms_metabolic, Template_relevance Pistachio_ringbreaker, Template_relevance Reaxys, Template_relevance Reaxys_biocatalysis model, leveraging a vast database of chemical reactions to predict feasible synthetic routes.
One-Step Synthesis Focus: Specifically designed for one-step synthesis, it provides concise and direct routes for your target compounds, streamlining the synthesis process.
Accurate Predictions: Utilizing the extensive PISTACHIO, BKMS_METABOLIC, PISTACHIO_RINGBREAKER, REAXYS, REAXYS_BIOCATALYSIS database, our tool offers high-accuracy predictions, reflecting the latest in chemical research and data.
Strategy Settings
Precursor scoring | Relevance Heuristic |
---|---|
Min. plausibility | 0.01 |
Model | Template_relevance |
Template Set | Pistachio/Bkms_metabolic/Pistachio_ringbreaker/Reaxys/Reaxys_biocatalysis |
Top-N result to add to graph | 6 |
Feasible Synthetic Routes
試験管内研究製品の免責事項と情報
BenchChemで提示されるすべての記事および製品情報は、情報提供を目的としています。BenchChemで購入可能な製品は、生体外研究のために特別に設計されています。生体外研究は、ラテン語の "in glass" に由来し、生物体の外で行われる実験を指します。これらの製品は医薬品または薬として分類されておらず、FDAから任何の医療状態、病気、または疾患の予防、治療、または治癒のために承認されていません。これらの製品を人間または動物に体内に導入する形態は、法律により厳格に禁止されています。これらのガイドラインに従うことは、研究と実験において法的および倫理的な基準の遵守を確実にするために重要です。