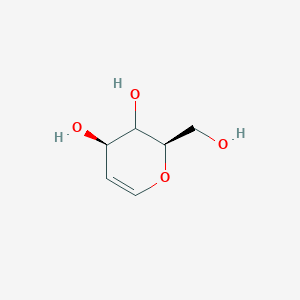
d-グルカル
概要
説明
d-Glucal: is a cyclic enol ether derivative of glucose, characterized by a double bond between the first and second carbon atoms of the ring. This compound is a member of the glycal family, which includes unsaturated sugar derivatives. d-Glucal was first synthesized by Hermann Emil Fischer and Karl Zach in 1913 from d-glucose . It has since become an important building block in synthetic carbohydrate chemistry due to its high reactivity and versatility.
科学的研究の応用
Chemistry:
Building Block: d-Glucal is used as a building block in the synthesis of complex carbohydrates and glycosides.
Glycosylation Donor: It is extensively used in glycosylation reactions to form oligosaccharides.
Biology and Medicine:
Enzyme Studies: d-Glucal and its derivatives are used to study enzyme mechanisms by selectively binding to amino acids in enzyme active sites.
Inhibitor: It has been shown to inhibit aflatoxin biosynthesis and promote kojic acid production in Aspergillus flavus.
Industry:
作用機序
Target of Action
d-Glucal is an organic compound belonging to the family of aldoses, which are monosaccharides containing an aldehyde functional group . It has been found to interact with various enzymes, including glucose oxidase and β-D-galactopyranosidase . These enzymes play crucial roles in metabolic processes, particularly in the breakdown and utilization of glucose.
Mode of Action
d-Glucal is a non-metabolizable glucose analog that inhibits certain biosynthetic pathways by suppressing the expression of specific biosynthetic genes . It interferes with glycolysis, a metabolic pathway that converts glucose into pyruvate, thereby disrupting the energy production process . Additionally, it can indirectly reduce oxidative stress through the promotion of kojic acid biosynthesis .
Biochemical Pathways
d-Glucal affects several biochemical pathways. It inhibits glycolysis, a fundamental pathway in cellular metabolism that breaks down glucose to produce energy . It also influences the biosynthesis of aflatoxin, a potent carcinogenic compound, by suppressing the expression of aflatoxin biosynthetic genes . Furthermore, it promotes the biosynthesis of kojic acid, a secondary metabolite with potential antioxidant properties .
Pharmacokinetics (ADME Properties)
Glucose is readily absorbed in the gut, widely distributed in the body, metabolized primarily in the liver, and excreted in urine
Result of Action
The molecular and cellular effects of d-Glucal’s action are primarily inhibitory. It suppresses the expression of certain biosynthetic genes, leading to the inhibition of specific metabolic pathways . This can result in reduced production of certain metabolites, such as aflatoxin . On the other hand, it promotes the production of kojic acid, potentially reducing oxidative stress .
Action Environment
The action, efficacy, and stability of d-Glucal can be influenced by various environmental factors. For instance, the presence of glucose in the environment can affect the inhibitory action of d-Glucal on glycolysis . Additionally, factors such as pH, temperature, and the presence of other metabolites can potentially influence the stability and efficacy of d-Glucal.
準備方法
Synthetic Routes and Reaction Conditions:
Reductive Elimination: The original synthesis of d-Glucal involved the reductive elimination of a glycosyl halide using zinc.
Ring-Closing Metathesis: Another method involves the ring-closing metathesis of suitable precursors to form the glycal structure.
Thioglycosides Reaction: The reaction of thioglycosides with lithium naphthalenide can also produce d-Glucal.
Mesylation and Beta-Elimination: Mesylation of the anomeric hydroxyl group followed by the formation of an anomeric palladium complex, which undergoes beta-elimination, is another synthetic route.
Industrial Production Methods: Industrial production of d-Glucal typically involves large-scale application of the above synthetic routes, optimized for yield and purity. The choice of method depends on the availability of starting materials and the desired scale of production.
化学反応の分析
Types of Reactions:
Electrophilic Addition:
Glycosylation: It is commonly used as a glycosylation donor in the synthesis of oligosaccharides.
Radical Reactions: Catalytic radical methods can functionalize the double bond of d-Glucal, providing access to a variety of glycoside derivatives.
Common Reagents and Conditions:
Electrophilic Addition: Halogens, epoxides, and nitrogen sources under appropriate conditions.
Glycosylation: Various glycosyl donors and acceptors in the presence of catalysts.
Radical Reactions: Radical initiators and catalysts under controlled conditions.
Major Products:
Electrophilic Addition: Halogenated, epoxidized, and nitrogen-substituted derivatives.
Glycosylation: Oligosaccharides and glycosides.
Radical Reactions: Functionalized glycoside derivatives.
類似化合物との比較
d-Galactal: Another glycal derived from galactose, which shares similar reactivity and applications.
d-Allal and d-Gulal: Glycals derived from other monosaccharides, used in similar synthetic applications.
Uniqueness: d-Glucal is unique due to its specific reactivity and the range of functional groups it can introduce into carbohydrate structures. Its ability to act as a glycosylation donor and its applications in enzyme studies and metabolic inhibition distinguish it from other glycals .
生物活性
d-Glucal, a glycal derived from glucose, has garnered attention in the field of medicinal chemistry due to its diverse biological activities and potential therapeutic applications. This article explores the biological activity of d-Glucal, focusing on its synthesis, mechanisms of action, and implications in cancer therapy, among other uses.
Overview of d-Glucal
d-Glucal is characterized by its unsaturated structure, which allows it to participate in various chemical reactions, making it a versatile building block in carbohydrate chemistry. Its unique properties enable the synthesis of numerous derivatives that exhibit significant biological activities.
Synthesis of d-Glucal Derivatives
The synthesis of d-Glucal derivatives is crucial for exploring their biological activities. Various synthetic pathways have been developed, including:
- Synthesis via Glycosylation Reactions : This method involves the reaction of d-Glucal with different nucleophiles to form glycosides. For instance, the reaction of d-Glucal with thiadiazole derivatives has been reported to yield compounds with promising anticancer properties .
- Modification through Cross-Coupling Reactions : Palladium-catalyzed cross-coupling reactions have been utilized to modify d-Glucal, leading to stable glycal boronates that can be further functionalized for enhanced biological activity .
- Formation of 2-Deoxy Derivatives : The conversion of d-Glucal into 2-deoxy derivatives has been extensively studied, resulting in compounds that exhibit altered biological profiles and improved pharmacological properties .
Anticancer Properties
d-Glucal and its derivatives have shown significant anticancer activity across various cancer cell lines. Notable findings include:
- Cytotoxic Effects : Studies have demonstrated that certain d-Glucal derivatives induce apoptosis in cancer cells such as MCF-7 (breast cancer) and HCT116 (colon cancer) through mechanisms involving cell cycle arrest and apoptosis induction .
- Pro-apoptotic Mechanisms : The introduction of specific functional groups onto the d-Glucal backbone enhances its ability to trigger apoptotic pathways in tumor cells, making it a candidate for further development as an anticancer agent .
Antiviral and Antimicrobial Activities
Research indicates that d-Glucal exhibits antiviral properties, particularly against viral infections where glucose metabolism plays a critical role. Its derivatives have been tested for efficacy against various pathogens:
- Antiviral Activity : Some studies suggest that d-Glucal can inhibit viral replication by interfering with the metabolic pathways essential for viral survival .
- Antimicrobial Effects : Derivatives of d-Glucal have shown promise as antimicrobial agents against both bacterial and fungal strains, highlighting their potential in treating infections resistant to conventional antibiotics .
Case Study 1: Anticancer Activity Assessment
A recent study synthesized several 2-deoxy derivatives from d-Glucal and evaluated their anticancer activity against various cell lines. The results indicated that certain derivatives exhibited enhanced cytotoxicity compared to their parent compounds, suggesting modifications to the glucal structure can significantly impact biological activity .
Compound | Cell Line Tested | IC50 (µM) | Mechanism |
---|---|---|---|
2-Deoxy-D-glucal Derivative A | MCF-7 | 25 | Apoptosis induction |
2-Deoxy-D-glucal Derivative B | HCT116 | 15 | Cell cycle arrest |
2-Deoxy-D-glucal Derivative C | HeLa | 20 | Apoptosis induction |
Case Study 2: Antiviral Efficacy
Another study investigated the antiviral properties of a novel derivative of d-Glucal against influenza virus. The compound demonstrated significant inhibition of viral replication in vitro, with a reported IC50 value much lower than that of standard antiviral drugs, indicating its potential as a therapeutic agent .
特性
IUPAC Name |
(2R,3S,4R)-2-(hydroxymethyl)-3,4-dihydro-2H-pyran-3,4-diol | |
---|---|---|
Source | PubChem | |
URL | https://pubchem.ncbi.nlm.nih.gov | |
Description | Data deposited in or computed by PubChem | |
InChI |
InChI=1S/C6H10O4/c7-3-5-6(9)4(8)1-2-10-5/h1-2,4-9H,3H2/t4-,5-,6+/m1/s1 | |
Source | PubChem | |
URL | https://pubchem.ncbi.nlm.nih.gov | |
Description | Data deposited in or computed by PubChem | |
InChI Key |
YVECGMZCTULTIS-PBXRRBTRSA-N | |
Source | PubChem | |
URL | https://pubchem.ncbi.nlm.nih.gov | |
Description | Data deposited in or computed by PubChem | |
Canonical SMILES |
C1=COC(C(C1O)O)CO | |
Source | PubChem | |
URL | https://pubchem.ncbi.nlm.nih.gov | |
Description | Data deposited in or computed by PubChem | |
Isomeric SMILES |
C1=CO[C@@H]([C@H]([C@@H]1O)O)CO | |
Source | PubChem | |
URL | https://pubchem.ncbi.nlm.nih.gov | |
Description | Data deposited in or computed by PubChem | |
Molecular Formula |
C6H10O4 | |
Record name | glucal | |
Source | Wikipedia | |
URL | https://en.wikipedia.org/wiki/Glucal | |
Description | Chemical information link to Wikipedia. | |
Source | PubChem | |
URL | https://pubchem.ncbi.nlm.nih.gov | |
Description | Data deposited in or computed by PubChem | |
DSSTOX Substance ID |
DTXSID60157685 | |
Record name | D-(+)-Glucal | |
Source | EPA DSSTox | |
URL | https://comptox.epa.gov/dashboard/DTXSID60157685 | |
Description | DSSTox provides a high quality public chemistry resource for supporting improved predictive toxicology. | |
Molecular Weight |
146.14 g/mol | |
Source | PubChem | |
URL | https://pubchem.ncbi.nlm.nih.gov | |
Description | Data deposited in or computed by PubChem | |
CAS No. |
13265-84-4 | |
Record name | D-(+)-Glucal | |
Source | CAS Common Chemistry | |
URL | https://commonchemistry.cas.org/detail?cas_rn=13265-84-4 | |
Description | CAS Common Chemistry is an open community resource for accessing chemical information. Nearly 500,000 chemical substances from CAS REGISTRY cover areas of community interest, including common and frequently regulated chemicals, and those relevant to high school and undergraduate chemistry classes. This chemical information, curated by our expert scientists, is provided in alignment with our mission as a division of the American Chemical Society. | |
Explanation | The data from CAS Common Chemistry is provided under a CC-BY-NC 4.0 license, unless otherwise stated. | |
Record name | D-(+)-Glucal | |
Source | EPA DSSTox | |
URL | https://comptox.epa.gov/dashboard/DTXSID60157685 | |
Description | DSSTox provides a high quality public chemistry resource for supporting improved predictive toxicology. | |
Record name | 1,5-anhydro-2-deoxy-D-arabino-hex-1-enitol | |
Source | European Chemicals Agency (ECHA) | |
URL | https://echa.europa.eu/substance-information/-/substanceinfo/100.032.949 | |
Description | The European Chemicals Agency (ECHA) is an agency of the European Union which is the driving force among regulatory authorities in implementing the EU's groundbreaking chemicals legislation for the benefit of human health and the environment as well as for innovation and competitiveness. | |
Explanation | Use of the information, documents and data from the ECHA website is subject to the terms and conditions of this Legal Notice, and subject to other binding limitations provided for under applicable law, the information, documents and data made available on the ECHA website may be reproduced, distributed and/or used, totally or in part, for non-commercial purposes provided that ECHA is acknowledged as the source: "Source: European Chemicals Agency, http://echa.europa.eu/". Such acknowledgement must be included in each copy of the material. ECHA permits and encourages organisations and individuals to create links to the ECHA website under the following cumulative conditions: Links can only be made to webpages that provide a link to the Legal Notice page. | |
Retrosynthesis Analysis
AI-Powered Synthesis Planning: Our tool employs the Template_relevance Pistachio, Template_relevance Bkms_metabolic, Template_relevance Pistachio_ringbreaker, Template_relevance Reaxys, Template_relevance Reaxys_biocatalysis model, leveraging a vast database of chemical reactions to predict feasible synthetic routes.
One-Step Synthesis Focus: Specifically designed for one-step synthesis, it provides concise and direct routes for your target compounds, streamlining the synthesis process.
Accurate Predictions: Utilizing the extensive PISTACHIO, BKMS_METABOLIC, PISTACHIO_RINGBREAKER, REAXYS, REAXYS_BIOCATALYSIS database, our tool offers high-accuracy predictions, reflecting the latest in chemical research and data.
Strategy Settings
Precursor scoring | Relevance Heuristic |
---|---|
Min. plausibility | 0.01 |
Model | Template_relevance |
Template Set | Pistachio/Bkms_metabolic/Pistachio_ringbreaker/Reaxys/Reaxys_biocatalysis |
Top-N result to add to graph | 6 |
Feasible Synthetic Routes
Q1: How is D-glucal structurally characterized using spectroscopic techniques?
A2: D-Glucal's structure is elucidated using various spectroscopic techniques, including NMR and MS. For instance, [1H NMR spectral data is often employed to confirm the structures of derivatives of functionalized C-β-D-glucopyranosides synthesized from D-glucal. []] Researchers studying the regiochemistry of D-glucal glucosylation utilized FAB MS and MALDI MS to confirm the monoglucosylated nature of the product. [] Additionally, CI MS comparisons with potential regioisomers helped definitively identify the reaction product as D-laminarabial. []
Q2: Does the choice of protecting groups affect the reactivity of D-glucal in Perlin reactions?
A3: Yes, research indicates that the choice of protecting groups significantly influences the outcome of Perlin reactions involving D-glucal. [] Specifically, benzyl and dimethoxyphenylmethyl protecting groups demonstrated compatibility with the reaction conditions, while several silyl protecting groups proved unsuitable. [] This highlights the importance of carefully selecting protecting groups to achieve desired reactivity in D-glucal transformations.
Q3: Can D-glucal act as a glycosyl donor in enzyme-catalyzed reactions?
A4: Yes, D-glucal can function as a glycosyl donor in the presence of certain enzymes. For instance, homogeneous endo-β-1,3-glucanase GA from Cellulomonas cellulans catalyzes the glycosylation of D-glucal using laminarin as the glycosyl residue donor. []
Q4: How do α-glucosidases and exo-α-glucanases differ in their stereochemical outcome when hydrating D-glucal?
A5: Studies revealed distinct stereochemical outcomes in D-glucal hydration depending on the enzyme employed. α-Glucosidases, sourced from various organisms, were found to protonate D-glucal from above its re face, resulting in 2-deoxy-D-glucose with α-configuration. [] In contrast, exo-α-glucanases (glucodextranase and glucoamylase) protonated D-glucal from below its si face. [] This difference highlights the varying mechanisms and active site geometries of these enzymes.
Q5: Can D-glucal participate in reactions catalyzed by alpha-glucan phosphorylases?
A6: Yes, D-glucal can substitute for glucose 1-phosphate as the glucosyl donor in reactions catalyzed by alpha-glucan phosphorylases from various sources. [] Notably, this reaction requires orthophosphate or arsenate and the enzyme must be in its active conformation with pyridoxal 5'-phosphate in its dianionic form. [] The reaction proceeds through a proposed mechanism involving protonation at C-2 of D-glucal, ultimately yielding 2-deoxy-alpha-D-glucose oligo- or polysaccharides. []
Q6: Can D-glucal be used to synthesize C-aryl glucals, and what are the advantages of different palladium-catalyzed methods?
A7: Yes, C-aryl glucals can be synthesized through palladium-catalyzed cross-coupling reactions using D-glucal derivatives. One approach involves coupling 1-tributylstannyl-3,4,6-tri-O-(tert-butyldimethylsilyl)-D-glucal with aryl bromides. [] Alternatively, 1-iodo-3,4,6-tri-O-(triisopropylsilyl)-D-glucal can be reacted with various metalated aromatics like ArZnCl, ArB(OH)2, and ArB(OMe)2. [] This latter method offers advantages such as higher yields, milder conditions, and a more efficient route to the iodo-glucal starting material. []
Q7: How does the structure of the fluorinating agent influence the stereochemical outcome in reactions with D-glucal?
A8: Research indicates that the choice of fluorinating agent significantly affects the stereoselectivity of D-glucal fluorination. For instance, reacting D-glucal with acetyl hypofluorite (AcOF) in a freon solvent (CFCl3) yields predominantly 2-deoxy-2-fluoro-D-glucose (2-FDG), achieving approximately 95% radiochemical purity. [] Conversely, using fluorine gas (F2) or AcOF in acetonitrile leads to the preferential formation of 2-deoxy-2-fluoro-D-mannose (2-FDM) over 2-FDG. [] These observations underscore the importance of carefully selecting the fluorinating agent and solvent to control the stereochemical outcome in D-glucal fluorination reactions.
Q8: Can D-glucal be utilized in the synthesis of 2-deoxy-α-d-glucopyranonucleosides?
A9: Yes, D-glucal serves as a valuable starting material for synthesizing 2-deoxy-α-d-glucopyranonucleosides. [] A key step in this process involves the halogen-mediated O-glycosidation of D-glucal using bromine in methanol. [] Subsequent steps include reductive removal of the halo group and hydrolysis of the methoxy group, ultimately yielding the desired 2-deoxy-d-glucose derivative. [] This approach highlights the versatility of D-glucal in accessing important nucleoside analogues.
Q9: How does the substitution at the C-3 position of D-glucal influence the stereoselectivity of chlorination reactions?
A10: The presence and nature of the substituent at the C-3 position of D-glucal significantly influence the stereochemical outcome of chlorination reactions. [] For example, chlorination of 3-O-acetyl-4,6-O-benzylidene-D-glucal favors β-side attack, leading to the predominant formation of the β-D-manno adduct. [] Conversely, chlorination of 3-deoxy-4,6-O-benzylidene-D-glucal under identical conditions primarily yields the β-D-arabino adduct. [] These findings underscore the importance of considering substituent effects on the stereoselectivity of reactions involving D-glucal derivatives.
Q10: Does the size of the macrocycle affect the biological activity of migrastatin analogues derived from D-glucal?
A11: Yes, the size of the macrocycle significantly influences the biological activity of migrastatin analogues synthesized from D-glucal. [] Studies comparing macrolactone, macrolactam, and macroketone analogues, all derived from D-glucal, revealed that a dorrigocin A analogue exhibited the most potent inhibitory activity against gastric cancer cell migration. [] This finding highlights the importance of exploring structural variations in the macrocycle for optimizing the biological activity of migrastatin analogues.
Q11: Can modifications to the olefin moiety in D-glucal derivatives influence the regioselectivity of hydrostannation reactions?
A12: Yes, the regioselectivity of palladium-catalyzed hydrostannation reactions involving D-glucal derivatives is influenced by modifications to the olefin moiety. [] Specifically, the presence of a neighboring acetyl group can induce chelation with the palladium catalyst, directing the stannyl group to add at a specific position. [] Additionally, the electronic nature of substituents on the alkene can also impact the regioselectivity of the reaction. [] This highlights the potential for fine-tuning the regiochemical outcome of D-glucal hydrostannation by modifying the olefin substituents.
Q12: Are polymers incorporating D-glucal units susceptible to degradation?
A13: Yes, polymers containing D-glucal units can exhibit susceptibility to degradation. For instance, copolymers of methyl methacrylate (MMA) and a D-glucal-derived cyclic ketene acetal (CKA) showed a tendency towards hydrolytic degradation. [] This susceptibility arises from the presence of ester linkages within the copolymer structure, originating from the ring-opening polymerization of the CKA monomer. []
Q13: Do copolymers of tri-O-acetyl-D-glucal and itaconic anhydride exhibit antimicrobial activity?
A14: Yes, research indicates that copolymers synthesized from tri-O-acetyl-D-glucal and itaconic anhydride demonstrate promising antimicrobial activity. [] The minimum microbicidal concentration-broth dilution method was used to evaluate the antimicrobial efficacy of these copolymers. [] The study found that both the homopolymers and copolymers of these monomers exhibited excellent antimicrobial properties. [] This discovery highlights the potential of these copolymers as novel antimicrobial agents.
Q14: What analytical techniques are used to characterize the products of D-glucal reactions?
A14: Various analytical techniques are employed to characterize the products of D-glucal reactions. These include:
- Nuclear Magnetic Resonance (NMR) Spectroscopy: Utilized to determine the structure, stereochemistry, and purity of D-glucal derivatives. [, , , , ]
- Mass Spectrometry (MS): Employed to confirm molecular weights and analyze fragmentation patterns of D-glucal-derived products. []
- X-ray Crystallography: Used to elucidate the three-dimensional structures of crystalline D-glucal derivatives, providing detailed information about bond lengths, angles, and conformations. [, ]
Q15: What is the potential for biodegradability of polymers incorporating D-glucal units?
A16: Polymers incorporating D-glucal units, particularly those with ester linkages, show promise for biodegradability. [] This characteristic stems from the inherent susceptibility of ester bonds to hydrolysis, making them amenable to breakdown in biological environments. []
試験管内研究製品の免責事項と情報
BenchChemで提示されるすべての記事および製品情報は、情報提供を目的としています。BenchChemで購入可能な製品は、生体外研究のために特別に設計されています。生体外研究は、ラテン語の "in glass" に由来し、生物体の外で行われる実験を指します。これらの製品は医薬品または薬として分類されておらず、FDAから任何の医療状態、病気、または疾患の予防、治療、または治癒のために承認されていません。これらの製品を人間または動物に体内に導入する形態は、法律により厳格に禁止されています。これらのガイドラインに従うことは、研究と実験において法的および倫理的な基準の遵守を確実にするために重要です。