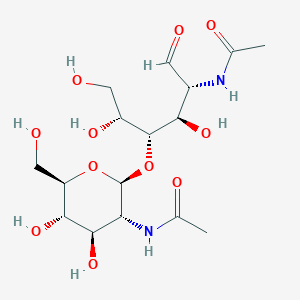
N,N'-diacetylchitobiose
説明
- Functionally Related to β-D-glucosaminyl-(1→4)-D-glucosamine : It is involved in the biosynthesis of this specific disaccharide .
Synthesis Analysis
N,N’-diacetylchitobiose can be produced via acid hydrolysis of chitin, but this method is costly and generates acidic waste. Alternatively, enzymatic degradation of chitin by chitinases leads to the formation of N,N’-diacetylchitobiose .
Molecular Structure Analysis
The molecular formula of N,N’-diacetylchitobiose is C₁₆H₂₈N₂O₁₁ , with a molecular weight of approximately 424.40 g/mol . It consists of two N-acetylglucosamine (GlcNAc) units linked by a β-(1→4) glycosidic bond . Here’s the structural depiction:
Chemical Reactions Analysis
N,N’-diacetylchitobiose is involved in chitin degradation pathways. It is produced from chitin by chitinases and serves as a substrate for enzymes like exo-β-D-glucosaminidase. The concerted action of these enzymes leads to the sequential deacetylation and hydrolysis of N,N’-diacetylchitobiose .
Physical And Chemical Properties Analysis
科学的研究の応用
Enzymatic Characterization
N,N’-Diacetylchitobiose is a primary substrate for the enzyme N,N’-Diacetylchitobiose Deacetylase from the extremophile Pyrococcus chitonophagus . This enzyme removes the acetyl group specifically from the non-reducing end of the sugar substrate . The enzyme is also active, at a reduced level, toward N-acetyl-d-glucosamine or a trimer of N-acetyl-d-glucosamine units .
Structural Analysis
The structure of the enzyme with its main substrate, N,N’-diacetylchitobiose, has been analyzed using X-ray crystallography . The crystallographic analysis includes the structure of the enzyme with its main substrate approaching the active site in a monodentate manner .
Thermodynamic Studies
The thermodynamics of the enzyme that uses N,N’-diacetylchitobiose as its main substrate has been studied using differential scanning calorimetry, isothermal titration calorimetry, and NMR . The enzyme is a hexameric, zinc-containing metalloenzyme that retains its structural integrity up to temperatures slightly exceeding 100 °C .
Antimicrobial Applications
N,N’-Diacetylchitobiose has been reported to possess antimicrobial activity against pathogenic bacteria . In a study, a 1% (w/v) solution of N,N’-Diacetylchitobiose was applied on ready-to-eat shrimp and evaluated as an antimicrobial coating against Listeria monocytogenes during storage at 4 °C .
Food Preservation
The same study also showed that the N,N’-Diacetylchitobiose coating was able to inhibit the growth of Listeria monocytogenes, while maintaining the quality of the ready-to-eat shrimp during refrigerated storage . This suggests potential applications of N,N’-Diacetylchitobiose in food preservation.
作用機序
Target of Action
N,N’-Diacetylchitobiose, a dimer of β(1,4) linked N-acetyl-D glucosamine, primarily targets the enzyme chitin deacetylase . This enzyme is key in the bioconversion of chitin to chitosan . Chitin deacetylase catalyzes the deacetylation of N-acetyl-D-glucosamine residues , which is an essential step in the degradation of chitin .
Mode of Action
N,N’-Diacetylchitobiose interacts with its target, chitin deacetylase, by serving as its main substrate . The enzyme removes the acetyl group specifically from the non-reducing end of the sugar substrate . While N,N’-Diacetylchitobiose is the main substrate, the enzyme is also active, at a reduced level, toward N-acetyl-D-glucosamine or a trimer of N-acetyl-D-glucosamine units .
Biochemical Pathways
The action of N,N’-Diacetylchitobiose affects the biochemical pathway of chitin degradation . Chitin deacetylase, the enzyme that N,N’-Diacetylchitobiose targets, plays a crucial role in this pathway. It catalyzes the deacetylation of N-acetyl-D-glucosamine residues, which is a key step in the degradation of chitin . This process results in the production of chitosan, a biopolymer with various industrial applications .
Pharmacokinetics
It is known that the compound is soluble in water , which could potentially influence its absorption, distribution, metabolism, and excretion (ADME) properties
Result of Action
The action of N,N’-Diacetylchitobiose results in the production of chitosan . Chitosan is a biopolymer with a wide array of applications in various industrial sectors, including pharmaceutics, gene delivery, tissue engineering, food and cosmetics industry, water treatment, and agriculture . The production of chitosan is a vital step towards its production in the realm of green chemistry .
Action Environment
The action of N,N’-Diacetylchitobiose is influenced by environmental factors. For instance, the enzyme chitin deacetylase, which N,N’-Diacetylchitobiose targets, retains its structural integrity up to temperatures slightly exceeding 100 °C This suggests that the action of N,N’-Diacetylchitobiose could be influenced by temperature
特性
IUPAC Name |
N-[(3R,4R,5S,6R)-5-[(2S,3R,4R,5S,6R)-3-acetamido-4,5-dihydroxy-6-(hydroxymethyl)oxan-2-yl]oxy-2,4-dihydroxy-6-(hydroxymethyl)oxan-3-yl]acetamide | |
---|---|---|
Source | PubChem | |
URL | https://pubchem.ncbi.nlm.nih.gov | |
Description | Data deposited in or computed by PubChem | |
InChI |
InChI=1S/C16H28N2O11/c1-5(21)17-9-13(25)14(8(4-20)27-15(9)26)29-16-10(18-6(2)22)12(24)11(23)7(3-19)28-16/h7-16,19-20,23-26H,3-4H2,1-2H3,(H,17,21)(H,18,22)/t7-,8-,9-,10-,11-,12-,13-,14-,15?,16+/m1/s1 | |
Source | PubChem | |
URL | https://pubchem.ncbi.nlm.nih.gov | |
Description | Data deposited in or computed by PubChem | |
InChI Key |
CDOJPCSDOXYJJF-CBTAGEKQSA-N | |
Source | PubChem | |
URL | https://pubchem.ncbi.nlm.nih.gov | |
Description | Data deposited in or computed by PubChem | |
Canonical SMILES |
CC(=O)NC1C(C(C(OC1O)CO)OC2C(C(C(C(O2)CO)O)O)NC(=O)C)O | |
Source | PubChem | |
URL | https://pubchem.ncbi.nlm.nih.gov | |
Description | Data deposited in or computed by PubChem | |
Isomeric SMILES |
CC(=O)N[C@@H]1[C@H]([C@@H]([C@H](O[C@H]1O[C@@H]2[C@H](OC([C@@H]([C@H]2O)NC(=O)C)O)CO)CO)O)O | |
Source | PubChem | |
URL | https://pubchem.ncbi.nlm.nih.gov | |
Description | Data deposited in or computed by PubChem | |
Molecular Formula |
C16H28N2O11 | |
Source | PubChem | |
URL | https://pubchem.ncbi.nlm.nih.gov | |
Description | Data deposited in or computed by PubChem | |
DSSTOX Substance ID |
DTXSID70956505 | |
Record name | 2-Deoxy-4-O-{2-deoxy-2-[(1-hydroxyethylidene)amino]hexopyranosyl}-2-[(1-hydroxyethylidene)amino]hexopyranose | |
Source | EPA DSSTox | |
URL | https://comptox.epa.gov/dashboard/DTXSID70956505 | |
Description | DSSTox provides a high quality public chemistry resource for supporting improved predictive toxicology. | |
Molecular Weight |
424.40 g/mol | |
Source | PubChem | |
URL | https://pubchem.ncbi.nlm.nih.gov | |
Description | Data deposited in or computed by PubChem | |
Product Name |
N,N'-diacetylchitobiose | |
CAS RN |
35061-50-8 | |
Record name | 2-Deoxy-4-O-{2-deoxy-2-[(1-hydroxyethylidene)amino]hexopyranosyl}-2-[(1-hydroxyethylidene)amino]hexopyranose | |
Source | EPA DSSTox | |
URL | https://comptox.epa.gov/dashboard/DTXSID70956505 | |
Description | DSSTox provides a high quality public chemistry resource for supporting improved predictive toxicology. | |
Record name | N,N'-Diacetylchitobiose | |
Source | European Chemicals Agency (ECHA) | |
URL | https://echa.europa.eu/information-on-chemicals | |
Description | The European Chemicals Agency (ECHA) is an agency of the European Union which is the driving force among regulatory authorities in implementing the EU's groundbreaking chemicals legislation for the benefit of human health and the environment as well as for innovation and competitiveness. | |
Explanation | Use of the information, documents and data from the ECHA website is subject to the terms and conditions of this Legal Notice, and subject to other binding limitations provided for under applicable law, the information, documents and data made available on the ECHA website may be reproduced, distributed and/or used, totally or in part, for non-commercial purposes provided that ECHA is acknowledged as the source: "Source: European Chemicals Agency, http://echa.europa.eu/". Such acknowledgement must be included in each copy of the material. ECHA permits and encourages organisations and individuals to create links to the ECHA website under the following cumulative conditions: Links can only be made to webpages that provide a link to the Legal Notice page. | |
Record name | N,N'-diacetylchitobiose | |
Source | Human Metabolome Database (HMDB) | |
URL | http://www.hmdb.ca/metabolites/HMDB0062702 | |
Description | The Human Metabolome Database (HMDB) is a freely available electronic database containing detailed information about small molecule metabolites found in the human body. | |
Explanation | HMDB is offered to the public as a freely available resource. Use and re-distribution of the data, in whole or in part, for commercial purposes requires explicit permission of the authors and explicit acknowledgment of the source material (HMDB) and the original publication (see the HMDB citing page). We ask that users who download significant portions of the database cite the HMDB paper in any resulting publications. | |
Retrosynthesis Analysis
AI-Powered Synthesis Planning: Our tool employs the Template_relevance Pistachio, Template_relevance Bkms_metabolic, Template_relevance Pistachio_ringbreaker, Template_relevance Reaxys, Template_relevance Reaxys_biocatalysis model, leveraging a vast database of chemical reactions to predict feasible synthetic routes.
One-Step Synthesis Focus: Specifically designed for one-step synthesis, it provides concise and direct routes for your target compounds, streamlining the synthesis process.
Accurate Predictions: Utilizing the extensive PISTACHIO, BKMS_METABOLIC, PISTACHIO_RINGBREAKER, REAXYS, REAXYS_BIOCATALYSIS database, our tool offers high-accuracy predictions, reflecting the latest in chemical research and data.
Strategy Settings
Precursor scoring | Relevance Heuristic |
---|---|
Min. plausibility | 0.01 |
Model | Template_relevance |
Template Set | Pistachio/Bkms_metabolic/Pistachio_ringbreaker/Reaxys/Reaxys_biocatalysis |
Top-N result to add to graph | 6 |
Feasible Synthetic Routes
試験管内研究製品の免責事項と情報
BenchChemで提示されるすべての記事および製品情報は、情報提供を目的としています。BenchChemで購入可能な製品は、生体外研究のために特別に設計されています。生体外研究は、ラテン語の "in glass" に由来し、生物体の外で行われる実験を指します。これらの製品は医薬品または薬として分類されておらず、FDAから任何の医療状態、病気、または疾患の予防、治療、または治癒のために承認されていません。これらの製品を人間または動物に体内に導入する形態は、法律により厳格に禁止されています。これらのガイドラインに従うことは、研究と実験において法的および倫理的な基準の遵守を確実にするために重要です。