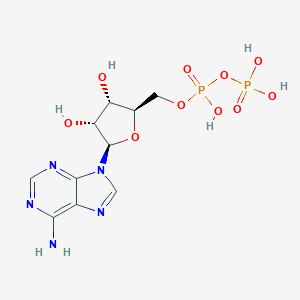
Adenosine-5'-diphosphate
説明
Adenosine-5'-diphosphate (ADP) is a nucleotide that plays a crucial role in energy metabolism in all living organisms. It is a precursor to adenosine triphosphate (ATP), which is the primary energy source for cellular processes. ADP is synthesized from adenosine monophosphate (AMP) and ATP through various enzymatic reactions.
科学的研究の応用
Enzymatic Assay Development
ADP plays a crucial role in biochemical and clinical chemistry assays. Techniques for estimating ADP and AMP (Adenosine-5'-monophosphate) involve enzymes like myokinase, pyruvate kinase, and lactate dehydrogenase in a single assay system. This method, based on the proportional decrease of NADH, offers simplicity and speed compared to chromatographic methods (Jaworek, Gruber, & Bergmeyer, 1974).
Inhibition Studies in Biochemistry
ADP has been identified as a competitive inhibitor of 5'-nucleotidase from rat heart, demonstrating a significant impact on enzymatic activity. Magnesium was found to relieve the inhibition imposed by ADP, highlighting the complex interplay between ADP and other biological molecules (Sullivan & Alpers, 1971).
Role in Cellular Metabolism and Stress Response
ADP is central to the functioning of poly(adenosine 5′-diphosphate) ribose polymerase, a nuclear enzyme that responds to DNA damage and stress. Its activation can trigger a severe energetic crisis in cells, impacting glycolysis and mitochondrial functions. This process is particularly relevant in conditions like systemic inflammation and ischemia-reperfusion (Liaudet, 2002).
Medical Imaging Techniques
ADP-based histochemical techniques have been developed to visualize human retinal vasculature. Adenosine diphosphatase, which hydrolyzes ADP, offers a novel approach for studying the vasculature in various conditions, providing insights into the structure and function of retinal blood vessels (Lutty & Mcleod, 1992).
ATP Regeneration in Biocatalytic Processes
Emerging research shows the potential of ADP in ATP regeneration systems, critical for enzymatic reactions. New methodologies are being developed to use ADP for efficient and cost-effective ATP regeneration, offering broad applications in biochemistry and biotechnology (Andexer & Richter, 2015).
Radioassays and Kinetic Studies
ADP is utilized in radioassays for studying enzyme activities, such as in human neutrophilic leukocyte homogenates. The development of these assays has helped understand the kinetic properties and function of specific enzymes (Smith, Smith, & Peters, 1980).
特性
IUPAC Name |
[(2R,3S,4R,5R)-5-(6-aminopurin-9-yl)-3,4-dihydroxyoxolan-2-yl]methyl phosphono hydrogen phosphate | |
---|---|---|
Source | PubChem | |
URL | https://pubchem.ncbi.nlm.nih.gov | |
Description | Data deposited in or computed by PubChem | |
InChI |
InChI=1S/C10H15N5O10P2/c11-8-5-9(13-2-12-8)15(3-14-5)10-7(17)6(16)4(24-10)1-23-27(21,22)25-26(18,19)20/h2-4,6-7,10,16-17H,1H2,(H,21,22)(H2,11,12,13)(H2,18,19,20)/t4-,6-,7-,10-/m1/s1 | |
Source | PubChem | |
URL | https://pubchem.ncbi.nlm.nih.gov | |
Description | Data deposited in or computed by PubChem | |
InChI Key |
XTWYTFMLZFPYCI-KQYNXXCUSA-N | |
Source | PubChem | |
URL | https://pubchem.ncbi.nlm.nih.gov | |
Description | Data deposited in or computed by PubChem | |
Canonical SMILES |
C1=NC(=C2C(=N1)N(C=N2)C3C(C(C(O3)COP(=O)(O)OP(=O)(O)O)O)O)N | |
Source | PubChem | |
URL | https://pubchem.ncbi.nlm.nih.gov | |
Description | Data deposited in or computed by PubChem | |
Isomeric SMILES |
C1=NC(=C2C(=N1)N(C=N2)[C@H]3[C@@H]([C@@H]([C@H](O3)COP(=O)(O)OP(=O)(O)O)O)O)N | |
Source | PubChem | |
URL | https://pubchem.ncbi.nlm.nih.gov | |
Description | Data deposited in or computed by PubChem | |
Molecular Formula |
C10H15N5O10P2 | |
Source | PubChem | |
URL | https://pubchem.ncbi.nlm.nih.gov | |
Description | Data deposited in or computed by PubChem | |
DSSTOX Substance ID |
DTXSID60883210 | |
Record name | Adenosine 5′-(trihydrogen diphosphate) | |
Source | EPA DSSTox | |
URL | https://comptox.epa.gov/dashboard/DTXSID60883210 | |
Description | DSSTox provides a high quality public chemistry resource for supporting improved predictive toxicology. | |
Molecular Weight |
427.20 g/mol | |
Source | PubChem | |
URL | https://pubchem.ncbi.nlm.nih.gov | |
Description | Data deposited in or computed by PubChem | |
Physical Description |
Solid | |
Record name | ADP | |
Source | Human Metabolome Database (HMDB) | |
URL | http://www.hmdb.ca/metabolites/HMDB0001341 | |
Description | The Human Metabolome Database (HMDB) is a freely available electronic database containing detailed information about small molecule metabolites found in the human body. | |
Explanation | HMDB is offered to the public as a freely available resource. Use and re-distribution of the data, in whole or in part, for commercial purposes requires explicit permission of the authors and explicit acknowledgment of the source material (HMDB) and the original publication (see the HMDB citing page). We ask that users who download significant portions of the database cite the HMDB paper in any resulting publications. | |
CAS RN |
58-64-0 | |
Record name | 5′-ADP | |
Source | CAS Common Chemistry | |
URL | https://commonchemistry.cas.org/detail?cas_rn=58-64-0 | |
Description | CAS Common Chemistry is an open community resource for accessing chemical information. Nearly 500,000 chemical substances from CAS REGISTRY cover areas of community interest, including common and frequently regulated chemicals, and those relevant to high school and undergraduate chemistry classes. This chemical information, curated by our expert scientists, is provided in alignment with our mission as a division of the American Chemical Society. | |
Explanation | The data from CAS Common Chemistry is provided under a CC-BY-NC 4.0 license, unless otherwise stated. | |
Record name | Adenosine diphosphate | |
Source | ChemIDplus | |
URL | https://pubchem.ncbi.nlm.nih.gov/substance/?source=chemidplus&sourceid=0000058640 | |
Description | ChemIDplus is a free, web search system that provides access to the structure and nomenclature authority files used for the identification of chemical substances cited in National Library of Medicine (NLM) databases, including the TOXNET system. | |
Record name | Adenosine disphosphate | |
Source | DrugBank | |
URL | https://www.drugbank.ca/drugs/DB16833 | |
Description | The DrugBank database is a unique bioinformatics and cheminformatics resource that combines detailed drug (i.e. chemical, pharmacological and pharmaceutical) data with comprehensive drug target (i.e. sequence, structure, and pathway) information. | |
Explanation | Creative Common's Attribution-NonCommercial 4.0 International License (http://creativecommons.org/licenses/by-nc/4.0/legalcode) | |
Record name | Adenosine 5'-(trihydrogen diphosphate) | |
Source | EPA Chemicals under the TSCA | |
URL | https://www.epa.gov/chemicals-under-tsca | |
Description | EPA Chemicals under the Toxic Substances Control Act (TSCA) collection contains information on chemicals and their regulations under TSCA, including non-confidential content from the TSCA Chemical Substance Inventory and Chemical Data Reporting. | |
Record name | Adenosine 5′-(trihydrogen diphosphate) | |
Source | EPA DSSTox | |
URL | https://comptox.epa.gov/dashboard/DTXSID60883210 | |
Description | DSSTox provides a high quality public chemistry resource for supporting improved predictive toxicology. | |
Record name | Adenosine 5'-(trihydrogen diphosphate) | |
Source | European Chemicals Agency (ECHA) | |
URL | https://echa.europa.eu/substance-information/-/substanceinfo/100.000.356 | |
Description | The European Chemicals Agency (ECHA) is an agency of the European Union which is the driving force among regulatory authorities in implementing the EU's groundbreaking chemicals legislation for the benefit of human health and the environment as well as for innovation and competitiveness. | |
Explanation | Use of the information, documents and data from the ECHA website is subject to the terms and conditions of this Legal Notice, and subject to other binding limitations provided for under applicable law, the information, documents and data made available on the ECHA website may be reproduced, distributed and/or used, totally or in part, for non-commercial purposes provided that ECHA is acknowledged as the source: "Source: European Chemicals Agency, http://echa.europa.eu/". Such acknowledgement must be included in each copy of the material. ECHA permits and encourages organisations and individuals to create links to the ECHA website under the following cumulative conditions: Links can only be made to webpages that provide a link to the Legal Notice page. | |
Record name | ADENOSINE DIPHOSPHATE | |
Source | FDA Global Substance Registration System (GSRS) | |
URL | https://gsrs.ncats.nih.gov/ginas/app/beta/substances/61D2G4IYVH | |
Description | The FDA Global Substance Registration System (GSRS) enables the efficient and accurate exchange of information on what substances are in regulated products. Instead of relying on names, which vary across regulatory domains, countries, and regions, the GSRS knowledge base makes it possible for substances to be defined by standardized, scientific descriptions. | |
Explanation | Unless otherwise noted, the contents of the FDA website (www.fda.gov), both text and graphics, are not copyrighted. They are in the public domain and may be republished, reprinted and otherwise used freely by anyone without the need to obtain permission from FDA. Credit to the U.S. Food and Drug Administration as the source is appreciated but not required. | |
Record name | ADP | |
Source | Human Metabolome Database (HMDB) | |
URL | http://www.hmdb.ca/metabolites/HMDB0001341 | |
Description | The Human Metabolome Database (HMDB) is a freely available electronic database containing detailed information about small molecule metabolites found in the human body. | |
Explanation | HMDB is offered to the public as a freely available resource. Use and re-distribution of the data, in whole or in part, for commercial purposes requires explicit permission of the authors and explicit acknowledgment of the source material (HMDB) and the original publication (see the HMDB citing page). We ask that users who download significant portions of the database cite the HMDB paper in any resulting publications. | |
Retrosynthesis Analysis
AI-Powered Synthesis Planning: Our tool employs the Template_relevance Pistachio, Template_relevance Bkms_metabolic, Template_relevance Pistachio_ringbreaker, Template_relevance Reaxys, Template_relevance Reaxys_biocatalysis model, leveraging a vast database of chemical reactions to predict feasible synthetic routes.
One-Step Synthesis Focus: Specifically designed for one-step synthesis, it provides concise and direct routes for your target compounds, streamlining the synthesis process.
Accurate Predictions: Utilizing the extensive PISTACHIO, BKMS_METABOLIC, PISTACHIO_RINGBREAKER, REAXYS, REAXYS_BIOCATALYSIS database, our tool offers high-accuracy predictions, reflecting the latest in chemical research and data.
Strategy Settings
Precursor scoring | Relevance Heuristic |
---|---|
Min. plausibility | 0.01 |
Model | Template_relevance |
Template Set | Pistachio/Bkms_metabolic/Pistachio_ringbreaker/Reaxys/Reaxys_biocatalysis |
Top-N result to add to graph | 6 |
Feasible Synthetic Routes
試験管内研究製品の免責事項と情報
BenchChemで提示されるすべての記事および製品情報は、情報提供を目的としています。BenchChemで購入可能な製品は、生体外研究のために特別に設計されています。生体外研究は、ラテン語の "in glass" に由来し、生物体の外で行われる実験を指します。これらの製品は医薬品または薬として分類されておらず、FDAから任何の医療状態、病気、または疾患の予防、治療、または治癒のために承認されていません。これらの製品を人間または動物に体内に導入する形態は、法律により厳格に禁止されています。これらのガイドラインに従うことは、研究と実験において法的および倫理的な基準の遵守を確実にするために重要です。