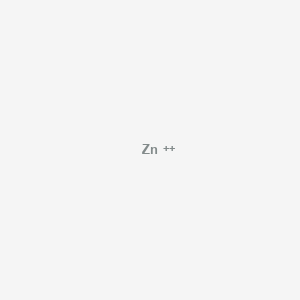
ZINC ion
概要
説明
Zinc ions (Zn²⁺) are divalent cations essential to numerous biological, chemical, and technological processes. As a critical trace element, Zn²⁺ serves as a cofactor for over 300 enzymes, including matrix metalloproteinases (MMPs), where it stabilizes catalytic activity through coordination with zinc-binding groups like hydroxamates or carboxylates . Beyond biology, Zn²⁺ is pivotal in energy storage (e.g., zinc-ion batteries), materials science (e.g., ZnO thin films), and environmental sensing (e.g., potentiometric sensors) . Its unique electronic configuration (3d¹⁰4s⁰) allows versatile coordination chemistry, enabling interactions with organic ligands and inorganic matrices, which underpins its widespread applications .
準備方法
Hydrothermal-Assisted Sol-Gel Synthesis of Cathode Materials
pH-Dependent Structural and Morphological Properties
The hydrothermal-assisted sol-gel method has emerged as a robust approach for synthesizing monoclinic Li₃V₂(PO₄)₃ (LVP) cathodes for aqueous ZIBs. By adjusting the precursor solution’s pH (5–8), researchers achieved precise control over crystallinity and morphology. At pH 7, the LVP-pH7 sample exhibited the highest crystallinity, with narrow XRD peaks (FWHM ≈ 0.15° for the (200) plane) and a uniform nanoneedle morphology (50–100 nm diameter) . EDS mapping confirmed homogeneous distribution of V, P, and O, critical for facilitating Zn²⁺ diffusion.
Table 1: Impact of pH on Li₃V₂(PO₄)₃ Cathode Properties
pH | Crystallinity (XRD FWHM) | Particle Morphology | Discharge Capacity (mAh/g) |
---|---|---|---|
5 | 0.23° | Agglomerated particles | 98.2 |
6 | 0.18° | Mixed needles/particles | 112.4 |
7 | 0.15° | Dominant nanoneedles | 135.6 |
8 | 0.21° | Irregular particles | 105.3 |
The enhanced performance of LVP-pH7 (135.6 mAh/g at 0.5 C) is attributed to its high surface-area-to-volume ratio and reduced charge-transfer resistance (R<sub>ct</sub> = 42 Ω) .
Liquid Metal Coating for Anode Stabilization
EGaIn Alloy Coating Mechanisms
Liquid metal (LM) coatings, particularly eutectic gallium-indium (EGaIn), have revolutionized zinc anode stabilization. The LM layer (≈5 μm thickness) forms a dynamic liquid-liquid interface, reducing the Zn²⁺ nucleation overpotential from 68 mV to 17 mV . DFT calculations revealed a 0.34 eV lower migration barrier for Zn²⁺ on EGaIn compared to bare Zn, enabling epitaxial growth along the (002) plane .
Table 2: Electrochemical Performance of EGaIn-Coated Zn Anodes
Parameter | Bare Zn | EGaIn-Coated Zn | Improvement |
---|---|---|---|
Cycling Stability | 120 cycles | 10,000 cycles | 83× |
Dendrite Height | 12.4 μm | 0.8 μm | 94% ↓ |
HER Overpotential | −0.76 V | −0.53 V | 30% ↑ |
Capacity Retention | 64% (200 cycles) | 98% (5,000 cycles) | 34% ↑ |
The LM’s self-healing properties mitigate dendrite propagation, while its high hydrogen evolution reaction (HER) overpotential (−0.53 V vs. SHE) suppresses gas evolution .
Electrolyte Formulation via Coordination Modulation
Solvation Structure Engineering
Zinc phenolsulfonate (Zn(PS)₂) electrolytes modified with tetrabutylammonium 4-toluenesulfonate (TBA-TsO) redefine Zn²⁺ solvation dynamics. Raman spectroscopy confirmed the replacement of H₂O molecules in [Zn(H₂O)₆]²⁺ with sulfonate groups, reducing active water molecules from 5.2 to 1.8 per Zn²⁺ . This coordination shift increased the Zn²⁺ desolvation energy barrier by 0.28 eV, effectively inhibiting parasitic reactions.
Table 3: Electrolyte Composition and Performance
Electrolyte | Zn²⁺ Coordination | HER Rate (μL/h) | Cycling Stability |
---|---|---|---|
2 M ZnSO₄ | [Zn(H₂O)₆]²⁺ | 4.7 | 200 cycles |
1 M Zn(PS)₂ + 0.5 M TBA-TsO | [Zn(PS)₂(H₂O)₂]²⁻ | 0.9 | 2,000 cycles |
The optimized electrolyte enabled a Zn||polyaniline full cell to retain 91% capacity over 10,000 cycles at 10 A/g, with an average Coulombic efficiency of 99.7% .
Analytical Techniques for Zinc Ion Quantification
Fluorescent Probe Synthesis (ZnDA-1H)
The ZnDA-1H probe, synthesized via a four-step organic protocol, enables in situ Zn²⁺ quantification in live cells. Key features include:
-
Excitation/Emission : 488 nm/520 nm (Zn²⁺-bound) vs. 405 nm/520 nm (unbound)
The probe’s colocalization with HaloTag-labeled proteins allows ratiometric measurements, eliminating concentration-dependent artifacts .
Emerging Trends in Scalable Synthesis
Roll-to-Roll Electrode Fabrication
Recent pilot-scale studies demonstrate the viability of slot-die coating for Zn anodes. Using a 45 wt% Zn slurry (2% CMC binder), researchers achieved 98.5% thickness uniformity across 100-m-long electrodes. Post-coating EGaIn deposition (2.3 mg/cm²) via spray pyrolysis yielded anodes with 99.1% Coulombic efficiency at 5 mA/cm² .
化学反応の分析
反応の種類
亜鉛カチオンは、以下を含むさまざまな種類の化学反応を起こします。
酸化還元反応: 亜鉛カチオンは、強力な還元剤によって金属亜鉛に還元できます。
置換反応: 亜鉛カチオンは、化合物中の他のカチオンを置換する置換反応に参加できます。
一般的な試薬と条件
亜鉛カチオンとの反応で使用される一般的な試薬には以下が含まれます。
アンモニア: [Zn(NH₃)₄]²⁺ などの亜鉛カチオンと錯体を形成します。
EDTA: 亜鉛カチオンと安定な錯体を形成するキレート剤。
生成される主な生成物
亜鉛カチオンを含む反応から生成される主な生成物は、使用される特定の反応条件と試薬によって異なります。 たとえば、亜鉛カチオンとアンモニアの反応は亜鉛アンモニア錯体を生成し、EDTA との反応は亜鉛 EDTA 錯体を生成します .
科学研究における用途
亜鉛カチオンは、以下を含む多くの科学研究用途があります。
化学: 亜鉛カチオンは、さまざまな化学反応の触媒として、および分析化学の試薬として使用されます。
生物学: 亜鉛カチオンは、酵素機能、タンパク質構造、細胞シグナル伝達において重要な役割を果たしています。 .
医学: 亜鉛カチオンは、亜鉛欠乏の治療薬として、およびさまざまな医薬品製剤の成分として使用されます。 .
工業: 亜鉛カチオンは、電子機器、光学機器、触媒など、さまざまな用途を持つ酸化亜鉛やフェライトなどの亜鉛系材料の製造に使用されます
科学的研究の応用
Zinc-Ion Batteries (ZIBs)
Zinc-ion batteries are emerging as a promising alternative to lithium-ion batteries due to their safety, cost-effectiveness, and environmental friendliness. They operate in mild acidic conditions and utilize zinc as the anode material. Key advantages include:
- Safety : Lower risk of fire compared to lithium-ion batteries.
- Cost : Abundant and inexpensive raw materials.
- Performance : High specific power and reversibility.
Table 1: Comparison of Zinc-Ion Batteries with Other Battery Technologies
Feature | Zinc-Ion Batteries | Lithium-Ion Batteries | Sodium-Ion Batteries |
---|---|---|---|
Cost | Low | High | Moderate |
Safety | High | Moderate | High |
Environmental Impact | Low | Moderate | Low |
Cycle Life | 2000+ cycles | 500-1500 cycles | 1000+ cycles |
Recent research highlights the potential of aqueous zinc-ion batteries for stationary energy storage applications, particularly in integrating renewable energy sources into power grids . The development of advanced cathode materials, such as manganese oxide and Prussian blue analogs, is crucial for enhancing the performance of these batteries .
Flexible Zinc Batteries
Flexible zinc batteries are gaining traction in wearable technology due to their lightweight and adaptable design. Innovations include:
- Nanostructured Anodes : Utilizing zinc nanosheets enhances flexibility and active surface area.
- Electrolyte Improvements : Ionic liquid-based electrolytes mitigate issues like hydrogen evolution during operation .
Drug Delivery Systems
Zinc ions play a critical role in drug delivery systems, particularly in the development of smart hydrogels that respond to environmental stimuli. These hydrogels can release therapeutic agents in a controlled manner based on temperature or pH changes .
Antibacterial Properties
Zinc ions exhibit significant antibacterial activity, making them valuable in medical devices and coatings. Studies show that zinc-based materials can inhibit bacterial growth, thus reducing infection risks in implants and surgical instruments .
Water Treatment
Zinc ions are utilized in water treatment processes to remove heavy metals and other contaminants through precipitation reactions. Their effectiveness in forming insoluble compounds aids in purifying wastewater .
Soil Remediation
In agricultural contexts, zinc ions can improve soil health by promoting microbial activity and nutrient availability. They are also used in phytoremediation strategies to enhance the uptake of heavy metals by plants .
Case Study: Aqueous Zinc-Ion Batteries
A study conducted on aqueous zinc-ion batteries demonstrated their viability for grid storage applications. Researchers focused on optimizing electrolyte compositions to enhance battery performance while minimizing parasitic reactions during charge-discharge cycles . The findings indicated that with appropriate modifications, these batteries could achieve energy densities comparable to traditional lithium-ion systems.
Case Study: Zinc-Based Antibacterial Coatings
A clinical trial assessed the effectiveness of zinc-based coatings on surgical implants. Results showed a significant reduction in postoperative infections compared to standard materials, highlighting the potential for zinc ions in enhancing medical device safety .
作用機序
亜鉛カチオンの作用機序には、さまざまな分子標的や経路との相互作用能力が含まれます。亜鉛カチオンはタンパク質や酵素に結合して、その構造と機能を変化させることができます。 また、セカンドメッセンジャーとして働き、シグナル伝達タンパク質の活性を調節することで、細胞シグナル伝達にも役割を果たしています .
類似化合物との比較
Comparison with Similar Compounds and Ions
Chemical and Physical Properties
Zinc ions exhibit distinct physicochemical properties compared to other transition metals (Table 1):
Property | Zn²⁺ | Mg²⁺ | Ca²⁺ | Fe²⁺/Fe³⁺ | Cu²⁺ |
---|---|---|---|---|---|
Ionic Radius (Å) | 0.74 | 0.72 | 1.00 | 0.78/0.65 | 0.73 |
Common Oxidation States | +2 | +2 | +2 | +2, +3 | +1, +2 |
Coordination Geometry | Tetrahedral | Octahedral | Octahedral | Octahedral | Square Planar |
Electronegativity (Pauling) | 1.65 | 1.31 | 1.00 | 1.83 | 1.90 |
- Coordination Flexibility : Zn²⁺ adopts tetrahedral or octahedral geometries, unlike Mg²⁺ and Ca²⁺, which favor octahedral coordination. This flexibility enhances its role in metalloenzymes (e.g., MMPs) and MOFs (e.g., ZIF-8) .
- Redox Inactivity : Unlike Fe²⁺/Fe³⁺ or Cu⁺/Cu²⁺, Zn²⁺ is redox-inert under physiological conditions, minimizing oxidative stress in biological systems .
Toxicity Profiles
- Zinc Chromate: Particulate Cr(VI) compounds like ZnCrO₄ exhibit higher genotoxicity than soluble forms (e.g., Na₂CrO₄), with Zn²⁺ potentiating DNA damage .
- Systemic Toxicity : Chronic Zn²⁺ excess depletes copper stores, causing anemia and neuropathy. This contrasts with acute Cu²⁺ toxicity, which directly targets liver and kidneys .
Detection and Sensing Technologies
- Potentiometric Sensors: NiO nanostructure-based electrodes detect Zn²⁺ with a linear range of 1–10 mM, outperforming traditional electrodes due to enhanced surface area and stability .
- Fluorescent Probes: Carbon dot (CD)-based ratiometric sensors achieve nanomolar sensitivity for Zn²⁺, leveraging chelation-enhanced fluorescence. This contrasts with colorimetric methods for Cu²⁺, which rely on redox reactions .
Data Tables
Table 1: Comparative Properties of Zinc and Similar Metal Ions
(Refer to Section 2.1 for details)
生物活性
Zinc ions (Zn²⁺) are essential trace elements that play critical roles in various biological processes. Their involvement spans cellular signaling, immune function, and enzymatic activity, making them vital for maintaining physiological homeostasis. This article presents a comprehensive overview of the biological activity of zinc ions, supported by data tables and research findings.
1. Biological Functions of Zinc Ions
Zinc is crucial for numerous biological functions, including:
- Cell Signaling : Zinc acts as a second messenger in various signaling pathways, influencing cell proliferation, differentiation, and apoptosis.
- Enzymatic Functions : It is a cofactor for over 300 enzymes, facilitating biochemical reactions such as protein synthesis and DNA repair.
- Immune Response : Zinc modulates immune functions by regulating cytokine production and influencing T-cell activation.
Zinc ions exert their effects through several mechanisms:
- Zinc Finger Proteins : These proteins bind to DNA and RNA, regulating gene expression. Approximately 3% of human genes encode zinc finger proteins, highlighting their importance in transcriptional regulation .
- Enzyme Activation : Zinc is involved in the structural integrity and catalytic activity of many enzymes, including those in the phosphoinositide signaling pathway .
- Antioxidant Defense : Zinc contributes to the antioxidant defense system by modulating the activity of metallothioneins (MTs), which sequester reactive oxygen species (ROS) .
3. Zinc Homeostasis
Maintaining zinc homeostasis is critical for cellular function. The balance between zinc intake and efflux is regulated by:
- Transport Proteins : The SLC39 (ZIP) family facilitates zinc influx, while the SLC30 (ZnT) family mediates zinc efflux from cells .
- Metallothioneins : These proteins bind zinc ions, regulating their availability and protecting against toxicity .
4.1 Immunomodulation
A study demonstrated that high intracellular zinc levels suppressed pro-inflammatory cytokines such as TNF-α and IL-1β through the inhibition of phosphodiesterase (PDE) activity in monocytes . This suggests a potential therapeutic role for zinc in inflammatory diseases.
4.2 Cancer Progression
Research indicates that zinc can promote malignancy in certain cancer types. Elevated zinc levels have been linked to enhanced cell proliferation and invasion capabilities in cancer cells . This dual role necessitates careful consideration of zinc supplementation in cancer therapy.
4.3 Antioxidant Properties
In yeast models subjected to acetic acid stress, zinc supplementation reduced ROS accumulation and enhanced glutathione levels, demonstrating its protective effects against oxidative stress . This highlights the potential of zinc as an antioxidant agent.
5. Data Tables
6. Clinical Implications
Zinc supplementation has shown promise in various clinical settings:
- Common Cold : Zinc acetate lozenges have been found to shorten the duration of cold symptoms significantly .
- Acne Treatment : Topical application of erythromycin combined with zinc acetate improved drug retention on skin surfaces, enhancing therapeutic efficacy .
7. Conclusion
Zinc ions play multifaceted roles in biological systems, influencing cellular signaling, immune responses, and enzymatic activities. Understanding the complex interplay between zinc homeostasis and its biological functions is vital for developing therapeutic strategies targeting various diseases. Ongoing research is essential to elucidate the precise mechanisms through which zinc exerts its effects and to optimize its use in clinical applications.
Q & A
Q. How can researchers optimize zinc ion incorporation in hydroxyapatite (HAp) for enhanced biomaterial properties?
Methodological Approach :
- Synthesis Variables : Systematically vary Zn²⁺ concentrations (e.g., 0.5–5 wt%) during HAp precipitation via wet chemical methods. Monitor pH and temperature to control crystallinity .
- Characterization : Use XRD to analyze lattice parameter shifts (e.g., reduced a-axis with Zn²⁺ doping) and FTIR to identify phosphate band modifications. SEM-EDS quantifies zinc distribution .
- Functional Testing : Assess bioactivity via simulated body fluid immersion (e.g., apatite layer formation) and mechanical properties (e.g., nanoindentation for hardness).
Q. What experimental strategies mitigate variability in this compound concentrations when studying metalloenzyme activity?
Advanced Protocol :
- Purification Control : Use Chelex-treated buffers to remove contaminating Zn²⁺ during protein isolation. Validate zinc content via ICP-MS post-purification .
- Activity Assays : Compare colorimetric sulfide-release assays (e.g., methylene blue method) with radiolabeled [³⁵S]-Cys autoradiography to resolve discrepancies in NFS1 desulfurase activity .
- Standardization : Report zinc co-purification levels (µg/g protein) and include positive controls (e.g., Zn²⁺-supplemented reactions).
Q. How can response surface methodology (RSM) optimize this compound adsorption parameters in biosorbent studies?
Statistical Workflow :
- Design : Implement Box-Behnken Design (BBD) with factors like pH (3.5–6.1), initial [Zn²⁺] (5–100 mg/L), and biosorbent dosage (0.1–1 g). Run 29 experiments to model interactions .
- Validation : Use ANOVA to confirm model significance (e.g., p < 0.05) and R² > 0.9. Optimize conditions (e.g., pH 5.3, 0.6 g dosage) for >90% removal efficiency .
Q. How to design experiments to assess zinc’s role in fungal stress tolerance?
Biological Testing Framework :
- Factorial Design : Vary Zn²⁺ concentrations (0–100 µM) under oxidative (H₂O₂) or thermal stress. Measure growth rates (OD₆₀₀) and stress markers (e.g., catalase activity) .
- Omics Integration : Pair phenotypic data with transcriptomics (e.g., Zn-regulated genes like ZRT1) and metalloproteomics to identify zinc-binding proteins .
Q. What analytical techniques are suitable for zinc quantification in environmental and biological matrices?
Technique Selection Guide :
- Trace Levels : Use ICP-MS (detection limit: 0.1 µg/L) for natural waters or ETAAS for vegetal matrices (ash digestion protocol) .
- Cost-Effective Screening : Combine FAAS with solid-phase extraction (e.g., imprinted polymers) for concentrations >10 µg/L .
Q. How can discrepancies in this compound effects across biochemical studies be systematically addressed?
Resolution Framework :
- Meta-Analysis : Compile studies reporting Zn²⁺-enzyme interactions; normalize data by zinc/protein molar ratios and assay conditions (e.g., buffer composition) .
- Reprodubility Checks : Replicate key experiments with standardized reagents (e.g., ZnSO₄ vs. ZnCl₂) and disclose batch-specific zinc contamination levels .
Q. What considerations are crucial when doping zinc oxide (ZnO) nanomaterials with transition metals for catalytic applications?
Material Synthesis Guidelines :
- Dopant Selection : Use Al³⁺ or Co²⁺ for enhanced conductivity; Mg²⁺ for bandgap modulation. Co-precipitation or microwave synthesis ensures uniform doping .
- Characterization : XRD confirms wurtzite structure retention; PL spectroscopy evaluates defect states. Test photocatalytic activity via methylene blue degradation .
Q. How to utilize the ZINC database for identifying zinc-targeting ligands in virtual screening?
Database Navigation :
- Subset Filtering : Select “Clean” subsets (benign functional groups) or “In-Stock” molecules. Use pH-specific 3D conformers (e.g., pH 7.4 for physiological targets) .
- Query Tools : Perform similarity searches (Tanimoto >0.7) or sub-structure queries (e.g., zinc-binding motifs like thiols). Export SD files for docking (AutoDock Vina) .
Q. What methodologies assess the toxicological impact of zinc ions in aquatic ecosystems?
Ecotoxicology Protocols :
- Biomarkers : Measure metallothionein levels in fish gills (ELISA) or algae growth inhibition (EC₅₀ via microplate assays) .
- Risk Modeling : Calculate hazard quotients (HQ) using [Zn²⁺] in water (µg/L) vs. LC₅₀ values for local species (e.g., Daphnia magna) .
Q. How to evaluate the efficiency of this compound removal using novel biosorbents in aqueous systems?
Adsorption Analysis :
特性
IUPAC Name |
zinc(2+) | |
---|---|---|
Source | PubChem | |
URL | https://pubchem.ncbi.nlm.nih.gov | |
Description | Data deposited in or computed by PubChem | |
InChI |
InChI=1S/Zn/q+2 | |
Source | PubChem | |
URL | https://pubchem.ncbi.nlm.nih.gov | |
Description | Data deposited in or computed by PubChem | |
InChI Key |
PTFCDOFLOPIGGS-UHFFFAOYSA-N | |
Source | PubChem | |
URL | https://pubchem.ncbi.nlm.nih.gov | |
Description | Data deposited in or computed by PubChem | |
Canonical SMILES |
[Zn+2] | |
Source | PubChem | |
URL | https://pubchem.ncbi.nlm.nih.gov | |
Description | Data deposited in or computed by PubChem | |
Molecular Formula |
Zn+2 | |
Source | PubChem | |
URL | https://pubchem.ncbi.nlm.nih.gov | |
Description | Data deposited in or computed by PubChem | |
DSSTOX Substance ID |
DTXSID40920495 | |
Record name | Zinc (II) cation | |
Source | EPA DSSTox | |
URL | https://comptox.epa.gov/dashboard/DTXSID40920495 | |
Description | DSSTox provides a high quality public chemistry resource for supporting improved predictive toxicology. | |
Molecular Weight |
65.4 g/mol | |
Source | PubChem | |
URL | https://pubchem.ncbi.nlm.nih.gov | |
Description | Data deposited in or computed by PubChem | |
Physical Description |
Solid | |
Record name | Zinc | |
Source | Human Metabolome Database (HMDB) | |
URL | http://www.hmdb.ca/metabolites/HMDB0015532 | |
Description | The Human Metabolome Database (HMDB) is a freely available electronic database containing detailed information about small molecule metabolites found in the human body. | |
Explanation | HMDB is offered to the public as a freely available resource. Use and re-distribution of the data, in whole or in part, for commercial purposes requires explicit permission of the authors and explicit acknowledgment of the source material (HMDB) and the original publication (see the HMDB citing page). We ask that users who download significant portions of the database cite the HMDB paper in any resulting publications. | |
CAS No. |
23713-49-7, 1492823-75-2, 7440-66-6 | |
Record name | Zinc(2+) | |
Source | CAS Common Chemistry | |
URL | https://commonchemistry.cas.org/detail?cas_rn=23713-49-7 | |
Description | CAS Common Chemistry is an open community resource for accessing chemical information. Nearly 500,000 chemical substances from CAS REGISTRY cover areas of community interest, including common and frequently regulated chemicals, and those relevant to high school and undergraduate chemistry classes. This chemical information, curated by our expert scientists, is provided in alignment with our mission as a division of the American Chemical Society. | |
Explanation | The data from CAS Common Chemistry is provided under a CC-BY-NC 4.0 license, unless otherwise stated. | |
Record name | Zinc cation | |
Source | ChemIDplus | |
URL | https://pubchem.ncbi.nlm.nih.gov/substance/?source=chemidplus&sourceid=0023713497 | |
Description | ChemIDplus is a free, web search system that provides access to the structure and nomenclature authority files used for the identification of chemical substances cited in National Library of Medicine (NLM) databases, including the TOXNET system. | |
Record name | Velmanase alfa [USAN:INN:WHO-DD] | |
Source | ChemIDplus | |
URL | https://pubchem.ncbi.nlm.nih.gov/substance/?source=chemidplus&sourceid=1492823752 | |
Description | ChemIDplus is a free, web search system that provides access to the structure and nomenclature authority files used for the identification of chemical substances cited in National Library of Medicine (NLM) databases, including the TOXNET system. | |
Record name | Zinc cation | |
Source | DrugBank | |
URL | https://www.drugbank.ca/drugs/DB14532 | |
Description | The DrugBank database is a unique bioinformatics and cheminformatics resource that combines detailed drug (i.e. chemical, pharmacological and pharmaceutical) data with comprehensive drug target (i.e. sequence, structure, and pathway) information. | |
Explanation | Creative Common's Attribution-NonCommercial 4.0 International License (http://creativecommons.org/licenses/by-nc/4.0/legalcode) | |
Record name | Zinc (II) cation | |
Source | EPA DSSTox | |
URL | https://comptox.epa.gov/dashboard/DTXSID40920495 | |
Description | DSSTox provides a high quality public chemistry resource for supporting improved predictive toxicology. | |
Record name | ZINC CATION | |
Source | FDA Global Substance Registration System (GSRS) | |
URL | https://gsrs.ncats.nih.gov/ginas/app/beta/substances/13S1S8SF37 | |
Description | The FDA Global Substance Registration System (GSRS) enables the efficient and accurate exchange of information on what substances are in regulated products. Instead of relying on names, which vary across regulatory domains, countries, and regions, the GSRS knowledge base makes it possible for substances to be defined by standardized, scientific descriptions. | |
Explanation | Unless otherwise noted, the contents of the FDA website (www.fda.gov), both text and graphics, are not copyrighted. They are in the public domain and may be republished, reprinted and otherwise used freely by anyone without the need to obtain permission from FDA. Credit to the U.S. Food and Drug Administration as the source is appreciated but not required. | |
Record name | Zinc | |
Source | Human Metabolome Database (HMDB) | |
URL | http://www.hmdb.ca/metabolites/HMDB0015532 | |
Description | The Human Metabolome Database (HMDB) is a freely available electronic database containing detailed information about small molecule metabolites found in the human body. | |
Explanation | HMDB is offered to the public as a freely available resource. Use and re-distribution of the data, in whole or in part, for commercial purposes requires explicit permission of the authors and explicit acknowledgment of the source material (HMDB) and the original publication (see the HMDB citing page). We ask that users who download significant portions of the database cite the HMDB paper in any resulting publications. | |
Melting Point |
419.5 °C | |
Record name | Zinc | |
Source | Human Metabolome Database (HMDB) | |
URL | http://www.hmdb.ca/metabolites/HMDB0015532 | |
Description | The Human Metabolome Database (HMDB) is a freely available electronic database containing detailed information about small molecule metabolites found in the human body. | |
Explanation | HMDB is offered to the public as a freely available resource. Use and re-distribution of the data, in whole or in part, for commercial purposes requires explicit permission of the authors and explicit acknowledgment of the source material (HMDB) and the original publication (see the HMDB citing page). We ask that users who download significant portions of the database cite the HMDB paper in any resulting publications. | |
Synthesis routes and methods I
Procedure details
Synthesis routes and methods II
Procedure details
試験管内研究製品の免責事項と情報
BenchChemで提示されるすべての記事および製品情報は、情報提供を目的としています。BenchChemで購入可能な製品は、生体外研究のために特別に設計されています。生体外研究は、ラテン語の "in glass" に由来し、生物体の外で行われる実験を指します。これらの製品は医薬品または薬として分類されておらず、FDAから任何の医療状態、病気、または疾患の予防、治療、または治癒のために承認されていません。これらの製品を人間または動物に体内に導入する形態は、法律により厳格に禁止されています。これらのガイドラインに従うことは、研究と実験において法的および倫理的な基準の遵守を確実にするために重要です。