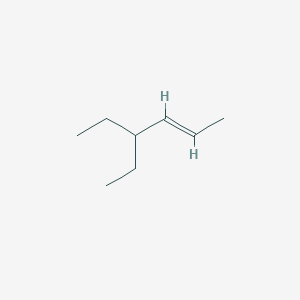
(E)-4-Ethylhex-2-ene
概要
説明
4-Ethyl-2-hexene is an organic compound with the molecular formula C₈H₁₆. It belongs to the class of alkenes, which are hydrocarbons containing at least one carbon-carbon double bond. This compound is characterized by the presence of an ethyl group attached to the second carbon of a hexene chain. Its structure can be represented as CH₃-CH₂-CH=CH-CH₂-CH₂-CH₃.
準備方法
Synthetic Routes and Reaction Conditions: 4-Ethyl-2-hexene can be synthesized through various methods, including:
Dehydration of Alcohols: One common method involves the dehydration of 4-ethyl-2-hexanol using a strong acid such as sulfuric acid. The reaction typically occurs at elevated temperatures to facilitate the removal of water and formation of the double bond.
Alkylation of Alkenes: Another method involves the alkylation of 1-hexene with ethyl chloride in the presence of a strong base like sodium amide. This reaction introduces the ethyl group at the desired position on the hexene chain.
Industrial Production Methods: Industrial production of 4-ethyl-2-hexene often involves catalytic processes to ensure high yield and purity. Catalysts such as zeolites or metal oxides are commonly used to facilitate the reaction and improve efficiency.
化学反応の分析
Types of Reactions: 4-Ethyl-2-hexene undergoes various chemical reactions, including:
Oxidation: This compound can be oxidized to form corresponding alcohols, aldehydes, or carboxylic acids. Common oxidizing agents include potassium permanganate and chromium trioxide.
Reduction: Hydrogenation of 4-ethyl-2-hexene in the presence of a metal catalyst like palladium or platinum results in the formation of 4-ethylhexane.
Substitution: Halogenation reactions, such as the addition of bromine, can occur at the double bond, leading to the formation of dihalogenated products.
Common Reagents and Conditions:
Oxidation: Potassium permanganate (KMnO₄) in an aqueous solution.
Reduction: Hydrogen gas (H₂) with a palladium catalyst.
Substitution: Bromine (Br₂) in an organic solvent like carbon tetrachloride.
Major Products Formed:
Oxidation: 4-ethyl-2-hexanol, 4-ethyl-2-hexanal, 4-ethyl-2-hexanoic acid.
Reduction: 4-ethylhexane.
Substitution: 4,5-dibromo-2-hexene.
科学的研究の応用
4-Ethyl-2-hexene has various applications in scientific research, including:
Chemistry: It is used as a starting material for the synthesis of more complex organic compounds. Its reactivity makes it a valuable intermediate in organic synthesis.
Medicine: Some derivatives of 4-ethyl-2-hexene are being investigated for their potential therapeutic properties, including anti-inflammatory and antimicrobial activities.
Industry: This compound is used in the production of specialty chemicals, including fragrances and flavorings, due to its unique structural properties.
作用機序
The mechanism of action of 4-ethyl-2-hexene in chemical reactions typically involves the interaction of the double bond with various reagents. For example, in electrophilic addition reactions, the double bond acts as a nucleophile, attacking electrophilic species such as halogens or hydrogen ions. This results in the formation of intermediate carbocations, which then undergo further reactions to yield the final products.
類似化合物との比較
4-Ethyl-2-hexene can be compared with other similar alkenes, such as:
4-Methyl-2-hexene: Similar in structure but with a methyl group instead of an ethyl group.
1-Hexene: Lacks the ethyl substituent, making it less sterically hindered.
2-Hexene: Similar chain length but without the ethyl group, leading to different reactivity and physical properties.
Uniqueness: The presence of the ethyl group in 4-ethyl-2-hexene introduces steric hindrance, which can influence its reactivity and selectivity in chemical reactions. This makes it a valuable compound for studying the effects of substituents on alkene reactivity.
生物活性
(E)-4-Ethylhex-2-ene, an alkene with the molecular formula C₈H₁₆, is a branched hydrocarbon characterized by a carbon-carbon double bond. This compound has garnered attention due to its potential applications in various fields, including organic synthesis and medicinal chemistry. This article delves into the biological activity of this compound, exploring its mechanisms, derivatives, and relevant research findings.
This compound is primarily known for its reactivity due to the presence of a double bond. The compound can undergo several chemical transformations:
- Electrophilic Addition Reactions : The double bond acts as a nucleophile, attacking electrophiles such as halogens, leading to the formation of carbocation intermediates. These intermediates can further react with nucleophiles to yield various products.
- Oxidation and Reduction : The compound can be oxidized to form alcohols and aldehydes or reduced to form alkanes. For instance, oxidation can yield 4-ethyl-2-hexanol or 4-ethyl-2-hexanal.
Biological Activity and Applications
While this compound itself is not commonly used directly in biological research, its derivatives have shown promising biological activities:
- Antimicrobial Properties : Some derivatives of this compound have been investigated for their antimicrobial activity. Research indicates that certain synthesized compounds exhibit significant antibacterial effects against pathogens like Escherichia coli and Staphylococcus aureus.
- Potential Therapeutic Uses : Derivatives are being explored for their potential anti-inflammatory and analgesic properties. Studies suggest that modifications to the alkene structure can enhance biological activity, making them candidates for drug development.
- Industrial Applications : Beyond medicinal chemistry, this compound is utilized in the production of specialty chemicals, including fragrances and flavorings, due to its unique structural properties.
Case Studies
Several studies highlight the biological implications of this compound and its derivatives:
Study 1: Antimicrobial Activity
A study synthesized various derivatives of this compound and tested their efficacy against common bacterial strains. Results indicated that specific compounds exhibited significant inhibition zones compared to control groups, suggesting potential as antimicrobial agents .
Study 2: Synthesis of Bioactive Compounds
Research focused on the synthesis of new bioactive compounds derived from this compound. These compounds were screened for their ability to interact with biological targets, revealing promising results in terms of selectivity and potency against certain diseases .
Data Summary
The following table summarizes key findings related to the biological activity of this compound derivatives:
Compound Type | Activity Type | Tested Microorganisms | Results |
---|---|---|---|
4-Ethylhexanamide | Antibacterial | E. coli, S. aureus | Significant inhibition observed |
4-Ethylhexanol | Anti-inflammatory | In vitro assays | Reduced inflammation markers |
Halogenated derivatives | Antimicrobial | Pseudomonas aeruginosa, Klebsiella pneumoniae | Moderate activity noted |
特性
IUPAC Name |
(E)-4-ethylhex-2-ene | |
---|---|---|
Source | PubChem | |
URL | https://pubchem.ncbi.nlm.nih.gov | |
Description | Data deposited in or computed by PubChem | |
InChI |
InChI=1S/C8H16/c1-4-7-8(5-2)6-3/h4,7-8H,5-6H2,1-3H3/b7-4+ | |
Source | PubChem | |
URL | https://pubchem.ncbi.nlm.nih.gov | |
Description | Data deposited in or computed by PubChem | |
InChI Key |
STHONQMAWQLWLX-QPJJXVBHSA-N | |
Source | PubChem | |
URL | https://pubchem.ncbi.nlm.nih.gov | |
Description | Data deposited in or computed by PubChem | |
Canonical SMILES |
CCC(CC)C=CC | |
Source | PubChem | |
URL | https://pubchem.ncbi.nlm.nih.gov | |
Description | Data deposited in or computed by PubChem | |
Isomeric SMILES |
CCC(CC)/C=C/C | |
Source | PubChem | |
URL | https://pubchem.ncbi.nlm.nih.gov | |
Description | Data deposited in or computed by PubChem | |
Molecular Formula |
C8H16 | |
Source | PubChem | |
URL | https://pubchem.ncbi.nlm.nih.gov | |
Description | Data deposited in or computed by PubChem | |
Molecular Weight |
112.21 g/mol | |
Source | PubChem | |
URL | https://pubchem.ncbi.nlm.nih.gov | |
Description | Data deposited in or computed by PubChem | |
CAS No. |
19780-46-2 | |
Record name | 4-Ethyl-2-hexene | |
Source | ChemIDplus | |
URL | https://pubchem.ncbi.nlm.nih.gov/substance/?source=chemidplus&sourceid=0019780462 | |
Description | ChemIDplus is a free, web search system that provides access to the structure and nomenclature authority files used for the identification of chemical substances cited in National Library of Medicine (NLM) databases, including the TOXNET system. | |
Retrosynthesis Analysis
AI-Powered Synthesis Planning: Our tool employs the Template_relevance Pistachio, Template_relevance Bkms_metabolic, Template_relevance Pistachio_ringbreaker, Template_relevance Reaxys, Template_relevance Reaxys_biocatalysis model, leveraging a vast database of chemical reactions to predict feasible synthetic routes.
One-Step Synthesis Focus: Specifically designed for one-step synthesis, it provides concise and direct routes for your target compounds, streamlining the synthesis process.
Accurate Predictions: Utilizing the extensive PISTACHIO, BKMS_METABOLIC, PISTACHIO_RINGBREAKER, REAXYS, REAXYS_BIOCATALYSIS database, our tool offers high-accuracy predictions, reflecting the latest in chemical research and data.
Strategy Settings
Precursor scoring | Relevance Heuristic |
---|---|
Min. plausibility | 0.01 |
Model | Template_relevance |
Template Set | Pistachio/Bkms_metabolic/Pistachio_ringbreaker/Reaxys/Reaxys_biocatalysis |
Top-N result to add to graph | 6 |
Feasible Synthetic Routes
試験管内研究製品の免責事項と情報
BenchChemで提示されるすべての記事および製品情報は、情報提供を目的としています。BenchChemで購入可能な製品は、生体外研究のために特別に設計されています。生体外研究は、ラテン語の "in glass" に由来し、生物体の外で行われる実験を指します。これらの製品は医薬品または薬として分類されておらず、FDAから任何の医療状態、病気、または疾患の予防、治療、または治癒のために承認されていません。これらの製品を人間または動物に体内に導入する形態は、法律により厳格に禁止されています。これらのガイドラインに従うことは、研究と実験において法的および倫理的な基準の遵守を確実にするために重要です。