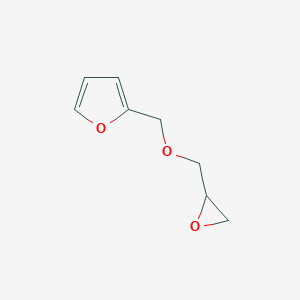
フルフリルグリシジルエーテル
概要
説明
Furfuryl glycidyl ether is an organic compound known for its unique properties and applications. It is a colorless liquid with a distinctive aldehyde-like odor. This compound is widely used in various fields, including resin synthesis, coatings, adhesives, rubber additives, and proton exchange membranes .
科学的研究の応用
Furfuryl glycidyl ether has numerous applications in scientific research:
準備方法
Synthetic Routes and Reaction Conditions: Furfuryl glycidyl ether is typically synthesized through a two-step process. First, furfuryl alcohol reacts with epichlorohydrin under basic conditions to form furfuryl glycidyl ether . The reaction involves the formation of an intermediate, which is then converted to the final product through a ring-opening reaction.
Industrial Production Methods: In industrial settings, furfuryl glycidyl ether is produced by reacting furfuryl alcohol with epichlorohydrin in the presence of a catalyst. The reaction is carried out under controlled temperature and pressure conditions to ensure high yield and purity .
化学反応の分析
Types of Reactions: Furfuryl glycidyl ether undergoes various chemical reactions, including:
Oxidation: It can be oxidized to form corresponding aldehydes and acids.
Reduction: Reduction reactions can convert it into alcohols.
Substitution: It can undergo nucleophilic substitution reactions, where the glycidyl group is replaced by other nucleophiles.
Common Reagents and Conditions:
Oxidation: Common oxidizing agents include potassium permanganate and chromium trioxide.
Reduction: Reducing agents such as lithium aluminum hydride and sodium borohydride are used.
Substitution: Nucleophiles like amines and thiols are commonly used in substitution reactions.
Major Products Formed:
Oxidation: Furfuryl aldehyde and furfuryl acid.
Reduction: Furfuryl alcohol.
Substitution: Various substituted furfuryl derivatives.
作用機序
The mechanism of action of furfuryl glycidyl ether involves its ability to undergo various chemical reactions, particularly the Diels-Alder reaction. This reaction allows it to form reversible covalent bonds with maleimides, leading to the creation of thermally reversible cross-linked polymers . These polymers exhibit enhanced mechanical properties and can be remolded and self-healed without loss of performance .
類似化合物との比較
- Furfuryl alcohol
- Furfural
- Furfurylamine
- Furfuryl methacrylate
Comparison: Furfuryl glycidyl ether stands out due to its unique ability to form reversible covalent bonds through the Diels-Alder reaction. This property makes it highly valuable in the synthesis of thermally reversible resins and self-healing materials . In contrast, similar compounds like furfuryl alcohol and furfural are primarily used as starting materials for the synthesis of other chemicals .
Furfuryl glycidyl ether’s versatility and unique properties make it a valuable compound in various scientific and industrial applications. Its ability to undergo a wide range of chemical reactions and form reversible covalent bonds sets it apart from other similar compounds.
生物活性
Furfuryl glycidyl ether (FGE) is a chemical compound that has garnered attention in various fields, particularly in biomedical applications due to its unique properties and biological activities. This article provides a comprehensive overview of the biological activity of FGE, including its synthesis, cytotoxicity, drug delivery capabilities, and case studies highlighting its applications in medical therapies.
1. Chemical Structure and Properties
Furfuryl glycidyl ether is characterized by its furan ring and glycidyl ether functional groups, which contribute to its reactivity and potential for forming copolymers. The synthesis typically involves the reaction of furfuryl alcohol with epichlorohydrin under basic conditions, yielding a compound with a molecular weight of approximately 138.16 g/mol.
2.1 Cytotoxicity Studies
Recent studies have demonstrated that FGE-based copolymers exhibit low cytotoxicity against various cell lines. For instance, a study on star-shaped poly(furfuryl glycidyl ether)-block-poly(glyceryl glycerol ether) (PFGE-b-PGGE) showed that even at high concentrations (100 µM), there was no significant decrease in the viability of human cervical cancer endothelial (HeLa) cells after 48 hours of incubation. The cell viability remained above 80%, indicating that these copolymers are non-cytotoxic and suitable for biomedical applications .
2.2 Drug Delivery Systems
Furfuryl glycidyl ether has been explored as a platform for drug delivery systems, particularly for hydrophobic drugs. The PFGE-b-PGGE copolymer was able to encapsulate nifuratel, a nitrofuran derivative used in gynecological therapies, with a high loading capacity of 146 mg per gram of copolymer. The release profile of nifuratel from these micelles demonstrated a diffusion-controlled mechanism, with approximately 60% of the drug released over 12 hours . This capability not only enhances the solubility of poorly soluble drugs but also minimizes toxicity to normal tissues.
3.1 Injectable and Self-Healable Hydrogels
Research has highlighted the application of FGE in developing injectable and self-healable hydrogels for medical use. The PFGE-b-PGGE copolymer forms hydrogels that can be injected into target areas and exhibit self-healing properties due to reversible cross-linking facilitated by furan moieties. These hydrogels can be utilized in tissue engineering and regenerative medicine, providing a scaffold that supports cell growth while delivering therapeutic agents .
3.2 Antimicrobial Activity
The antimicrobial properties of FGE derivatives have also been investigated. Nifuratel-loaded PFGE-b-PGGE micelles have shown broad-spectrum antimicrobial activity against pathogens responsible for vulvovaginitis and candidiasis, making them promising candidates for treating gynecological infections .
4. Comparative Analysis
The following table summarizes key findings related to the biological activity of furfuryl glycidyl ether-based compounds:
Property | PFGE-b-PGGE | Traditional Drug Delivery Systems |
---|---|---|
Cytotoxicity | Non-cytotoxic (≥80% viability at 100 µM) | Varies; often higher toxicity |
Drug Loading Capacity | 146 mg nifuratel/g copolymer | Typically lower |
Release Mechanism | Diffusion-controlled | Often burst release |
Self-Healing Properties | Yes | Rarely present |
Antimicrobial Activity | Broad-spectrum | Limited to specific formulations |
5. Conclusion
Furfuryl glycidyl ether presents significant potential in biomedical applications due to its low cytotoxicity, effective drug delivery capabilities, and versatility in forming advanced materials like self-healing hydrogels. Ongoing research continues to explore its applications in enhancing therapeutic efficacy while minimizing side effects, particularly in gynecological treatments.
The findings indicate that FGE not only serves as a valuable building block for novel polymeric systems but also plays a crucial role in advancing drug delivery technologies aimed at improving patient outcomes in various medical fields. Further studies are warranted to explore additional applications and optimize formulations for clinical use.
特性
IUPAC Name |
2-(oxiran-2-ylmethoxymethyl)furan | |
---|---|---|
Source | PubChem | |
URL | https://pubchem.ncbi.nlm.nih.gov | |
Description | Data deposited in or computed by PubChem | |
InChI |
InChI=1S/C8H10O3/c1-2-7(10-3-1)4-9-5-8-6-11-8/h1-3,8H,4-6H2 | |
Source | PubChem | |
URL | https://pubchem.ncbi.nlm.nih.gov | |
Description | Data deposited in or computed by PubChem | |
InChI Key |
RUGWIVARLJMKDM-UHFFFAOYSA-N | |
Source | PubChem | |
URL | https://pubchem.ncbi.nlm.nih.gov | |
Description | Data deposited in or computed by PubChem | |
Canonical SMILES |
C1C(O1)COCC2=CC=CO2 | |
Source | PubChem | |
URL | https://pubchem.ncbi.nlm.nih.gov | |
Description | Data deposited in or computed by PubChem | |
Molecular Formula |
C8H10O3 | |
Source | PubChem | |
URL | https://pubchem.ncbi.nlm.nih.gov | |
Description | Data deposited in or computed by PubChem | |
DSSTOX Substance ID |
DTXSID90968589 | |
Record name | 2-{[(Oxiran-2-yl)methoxy]methyl}furan | |
Source | EPA DSSTox | |
URL | https://comptox.epa.gov/dashboard/DTXSID90968589 | |
Description | DSSTox provides a high quality public chemistry resource for supporting improved predictive toxicology. | |
Molecular Weight |
154.16 g/mol | |
Source | PubChem | |
URL | https://pubchem.ncbi.nlm.nih.gov | |
Description | Data deposited in or computed by PubChem | |
CAS No. |
5380-87-0 | |
Record name | 2-[(2-Oxiranylmethoxy)methyl]furan | |
Source | CAS Common Chemistry | |
URL | https://commonchemistry.cas.org/detail?cas_rn=5380-87-0 | |
Description | CAS Common Chemistry is an open community resource for accessing chemical information. Nearly 500,000 chemical substances from CAS REGISTRY cover areas of community interest, including common and frequently regulated chemicals, and those relevant to high school and undergraduate chemistry classes. This chemical information, curated by our expert scientists, is provided in alignment with our mission as a division of the American Chemical Society. | |
Explanation | The data from CAS Common Chemistry is provided under a CC-BY-NC 4.0 license, unless otherwise stated. | |
Record name | Furfuryl glycidyl ether | |
Source | ChemIDplus | |
URL | https://pubchem.ncbi.nlm.nih.gov/substance/?source=chemidplus&sourceid=0005380870 | |
Description | ChemIDplus is a free, web search system that provides access to the structure and nomenclature authority files used for the identification of chemical substances cited in National Library of Medicine (NLM) databases, including the TOXNET system. | |
Record name | 2-{[(Oxiran-2-yl)methoxy]methyl}furan | |
Source | EPA DSSTox | |
URL | https://comptox.epa.gov/dashboard/DTXSID90968589 | |
Description | DSSTox provides a high quality public chemistry resource for supporting improved predictive toxicology. | |
Record name | 2-[(2,3-epoxypropoxy)methyl]furan | |
Source | European Chemicals Agency (ECHA) | |
URL | https://echa.europa.eu/substance-information/-/substanceinfo/100.023.974 | |
Description | The European Chemicals Agency (ECHA) is an agency of the European Union which is the driving force among regulatory authorities in implementing the EU's groundbreaking chemicals legislation for the benefit of human health and the environment as well as for innovation and competitiveness. | |
Explanation | Use of the information, documents and data from the ECHA website is subject to the terms and conditions of this Legal Notice, and subject to other binding limitations provided for under applicable law, the information, documents and data made available on the ECHA website may be reproduced, distributed and/or used, totally or in part, for non-commercial purposes provided that ECHA is acknowledged as the source: "Source: European Chemicals Agency, http://echa.europa.eu/". Such acknowledgement must be included in each copy of the material. ECHA permits and encourages organisations and individuals to create links to the ECHA website under the following cumulative conditions: Links can only be made to webpages that provide a link to the Legal Notice page. | |
Synthesis routes and methods
Procedure details
Retrosynthesis Analysis
AI-Powered Synthesis Planning: Our tool employs the Template_relevance Pistachio, Template_relevance Bkms_metabolic, Template_relevance Pistachio_ringbreaker, Template_relevance Reaxys, Template_relevance Reaxys_biocatalysis model, leveraging a vast database of chemical reactions to predict feasible synthetic routes.
One-Step Synthesis Focus: Specifically designed for one-step synthesis, it provides concise and direct routes for your target compounds, streamlining the synthesis process.
Accurate Predictions: Utilizing the extensive PISTACHIO, BKMS_METABOLIC, PISTACHIO_RINGBREAKER, REAXYS, REAXYS_BIOCATALYSIS database, our tool offers high-accuracy predictions, reflecting the latest in chemical research and data.
Strategy Settings
Precursor scoring | Relevance Heuristic |
---|---|
Min. plausibility | 0.01 |
Model | Template_relevance |
Template Set | Pistachio/Bkms_metabolic/Pistachio_ringbreaker/Reaxys/Reaxys_biocatalysis |
Top-N result to add to graph | 6 |
Feasible Synthetic Routes
Q1: What is the molecular formula and weight of FGE?
A1: FGE has a molecular formula of C8H10O3 and a molecular weight of 154.16 g/mol.
Q2: What spectroscopic data is available for FGE?
A2: FGE can be characterized using techniques like Fourier transform infrared spectroscopy (FTIR) and nuclear magnetic resonance (1H NMR) spectroscopy. FTIR helps identify functional groups, while 1H NMR provides insights into the arrangement of hydrogen atoms within the molecule. []
Q3: What makes FGE attractive for material applications?
A3: FGE possesses a unique combination of a reactive epoxy group and a furan ring. The epoxy group allows for polymerization and crosslinking reactions, while the furan ring enables reversible modifications via Diels-Alder chemistry. [, , ]
Q4: How does the presence of the furan ring in FGE contribute to its material properties?
A4: The furan ring in FGE enables the creation of thermally reversible crosslinked networks through Diels-Alder reactions with maleimide-containing compounds. This reversibility is crucial for developing self-healing materials. [, , , , , ]
Q5: What is the significance of using FGE in synthesizing poly(propylene carbonate) (PPC)?
A5: FGE, when copolymerized with carbon dioxide and propylene oxide, introduces cross-linking points into the PPC structure. This enhances the thermal and dimensional stability of the resulting copolymer, addressing the brittleness often associated with PPC. [, ]
Q6: How does FGE contribute to the development of self-healing epoxy resins?
A6: FGE acts as a reactive diluent and introduces furan functionality within the epoxy resin network. Upon reaction with bismaleimides, thermally reversible Diels-Alder crosslinks are formed, enabling the resin to self-heal at elevated temperatures. [, , , ]
Q7: Can FGE be used to modify the properties of existing polymers?
A7: Yes, FGE can be incorporated into various polymer systems. For example, it can be used to modify polysulfone (PSU) to improve its crack resistance by creating a thermoplastic-enriched interpenetrating network. []
Q8: What role does FGE play in forming core-crosslinked micelles?
A8: FGE can be incorporated into amphiphilic block copolymers, forming the hydrophobic core of micelles in aqueous solutions. The furan rings in the core can be crosslinked using bismaleimides via Diels-Alder chemistry, enhancing the micelle's stability. [, , ]
Q9: Can FGE be used to create stimuli-responsive materials?
A9: Yes, by incorporating FGE into polymer networks, stimuli-responsive hydrogels can be created. For instance, FGE-modified gelatin hydrogels crosslinked with bismaleimides exhibited pH and salt-responsive swelling behavior. []
Q10: How does FGE contribute to the development of biocompatible materials?
A10: FGE has been successfully used to modify biopolymers like gelatin and chitosan. The furan functionality allows for further modification, potentially creating biocompatible and biodegradable materials for biomedical applications. [, ]
Q11: How is FGE synthesized?
A11: FGE is typically synthesized via the reaction of furfuryl alcohol with epichlorohydrin. This reaction can be facilitated using various catalysts, and the choice of catalyst influences the reaction conditions and purity of the FGE product. [, ]
Q12: How can the molecular weight of FGE-containing polymers be controlled?
A12: Techniques like living anionic ring-opening polymerization (AROP) offer precise control over molecular weight and dispersity of FGE-containing polymers. This control is vital for tailoring material properties. [, , ]
Q13: What analytical methods are used to characterize FGE and its derivatives?
A13: Alongside FTIR and 1H NMR, techniques like size exclusion chromatography (SEC), differential scanning calorimetry (DSC), and thermogravimetric analysis (TGA) are employed to determine molecular weight distribution, thermal transitions, and degradation profiles, respectively. [, , , , ]
Q14: Can computational methods be used to study FGE and its polymers?
A14: Yes, molecular dynamics (MD) simulations provide valuable insights into the structure-property relationships of FGE-containing polymers. These simulations help predict and optimize material properties based on their molecular composition. []
Q15: What makes FGE a promising candidate for sustainable material development?
A15: FGE can be derived from renewable resources like furfural, a byproduct of biomass processing. Utilizing FGE in polymer synthesis aligns with the growing emphasis on sustainable and bio-based materials. [, ]
Q16: What are potential future directions for FGE research?
A16: Further exploration of FGE in drug delivery systems, shape-memory materials, and stimuli-responsive coatings hold promise. Continued research on its biocompatibility and biodegradability is crucial for advancing biomedical applications. [, , , , ]
Q17: What are the limitations of current FGE research?
A17: While promising, FGE research is still developing. Further investigations on long-term stability, mechanical properties under various conditions, and potential environmental impact are needed. [, ]
試験管内研究製品の免責事項と情報
BenchChemで提示されるすべての記事および製品情報は、情報提供を目的としています。BenchChemで購入可能な製品は、生体外研究のために特別に設計されています。生体外研究は、ラテン語の "in glass" に由来し、生物体の外で行われる実験を指します。これらの製品は医薬品または薬として分類されておらず、FDAから任何の医療状態、病気、または疾患の予防、治療、または治癒のために承認されていません。これらの製品を人間または動物に体内に導入する形態は、法律により厳格に禁止されています。これらのガイドラインに従うことは、研究と実験において法的および倫理的な基準の遵守を確実にするために重要です。