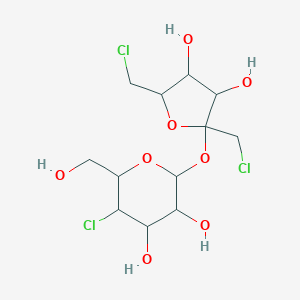
スクラロース
概要
説明
Sucralose, with the chemical formula C12H19Cl3O8, is a high-intensity sweetener. It was jointly developed by the British company Tate & Lyle and the University of London, with a patent application filed in 1976 . Unlike other artificial sweeteners, sucralose is unique because it is derived from sucrose (table sugar). Its original brand name, Splenda, reflects its remarkable sweetness—approximately 320 to 1000 times sweeter than sucrose.
科学的研究の応用
Food and Beverage Industry
Sucralose is predominantly used as a sweetener in a wide range of food products, including:
- Beverages : Soft drinks, flavored waters, and energy drinks.
- Baked Goods : Cakes, cookies, and pastries.
- Dairy Products : Yogurts and ice creams.
- Condiments : Ketchup and salad dressings.
The ability of sucralose to maintain sweetness during cooking and baking makes it a preferred choice for manufacturers aiming to reduce caloric content without sacrificing taste.
Metabolic Research
Recent studies have examined the effects of sucralose on metabolic health, particularly its influence on insulin secretion and glucose metabolism:
- Insulin Response : Research indicates that sucralose can enhance insulin secretion in response to glucose. For instance, studies using isolated mouse pancreatic islets demonstrated that sucralose activates sweet taste receptors (TAS1R2/TAS1R3), leading to increased insulin release when glucose is present .
- Glycemic Control : Some investigations have suggested that regular consumption of sucralose may modulate glycemic responses. A study found that sucralose intake was associated with elevated glucose absorption in rodent models .
Impact on Gut Microbiota
Emerging evidence suggests that artificial sweeteners like sucralose may alter gut microbiota composition. A study highlighted that consuming sucralose could lead to significant changes in microbial communities within the duodenum, potentially impacting overall metabolic health . This area remains under investigation as researchers seek to understand the long-term implications of such alterations.
Immunomodulatory Effects
Recent findings have indicated that high doses of sucralose may exert immunomodulatory effects by influencing T cell function:
- T Cell Proliferation : In vitro studies showed that sucralose could limit T cell proliferation and differentiation, potentially affecting immune responses in models of autoimmunity and cancer .
- Therapeutic Potential : These effects raise intriguing possibilities for using sucralose in therapeutic contexts where modulation of immune responses is beneficial.
Safety Profile and Regulatory Status
Sucralose has been extensively studied for safety and is generally recognized as safe (GRAS) by regulatory bodies such as the FDA and EFSA. It has been deemed safe for consumption across various demographics, including pregnant women and children . The acceptable daily intake (ADI) established by these organizations provides guidelines for safe consumption levels.
Case Studies
作用機序
スクラロースは体内で代謝されず、変化せずに排泄されます。スクラロースは舌の味覚受容体に作用し、カロリーを摂取することなく甘味を提供します。 スクラロースの安全性は広く評価されており、推奨される範囲内で摂取する場合、安全であると考えられています .
6. 類似化合物の比較
スクラロースは、スクロース由来で、甘味が強いという点で際立っています。その他の類似化合物には、アスパルテーム、サッカリン、ステビア配糖体などがあります。
生化学分析
Biochemical Properties
Sucralose interacts with chemosensors in the alimentary tract that play a role in sweet taste sensation and hormone secretion . In rats, sucralose ingestion was shown to increase the expression of the efflux transporter P-glycoprotein (P-gp) and two cytochrome P-450 (CYP) isozymes in the intestine . P-gp and CYP are key components of the presystemic detoxification system involved in first-pass drug metabolism .
Cellular Effects
Research done on animals shows that sucralose can increase inflammation in the body . Over time, inflammation can lead to problems like obesity and diabetes . Sucralose has also been found to affect the proliferation and differentiation of T cells . No effect was observed for B cells and myeloid cells .
Molecular Mechanism
Sucralose interferes with T-cell membrane order and limits PLCγ1-dependent induction of intracellular calcium release into the cytoplasm . Several mechanisms by which sucralose might potentially affect lipid raft formation and/or targeting of individual proteins to lipid rafts are conceivable .
Temporal Effects in Laboratory Settings
The temporal profile of sucralose is different from other sweeteners, with a greater persistence of sweetness in the mouth . The evaluation of the sweeteners in yogurt by consumers using Temporal Dominance of Sensations (TDS) and temporal acceptability method showed that stevia and aspartame showed higher acceptability than other sweeteners .
Dosage Effects in Animal Models
High doses of sucralose reduce immune responses in mice . The researchers did not investigate the sweetener’s effects in humans, and say that it is unlikely that normal consumption of sucralose is harmful .
Metabolic Pathways
Sucralose is a food additive initially used to mitigate glycemic peaks and calorie intake in patients with diabetes and obesity . This review aims to comprehensively explore the effects of sucralose intake on human health by understanding sucralose absorption, metabolism, and excretion .
Transport and Distribution
The transportation of sucrose is divided into active transportation and passive transportation . The long distance is in the concept of “source”, “sink” and “transportation” .
Subcellular Localization
Recent advances on the subcellular localisation and function of sucrose transporters, sucrose facilitators and sucrose transporter-like proteins open new questions about the role of membrane compartmentation on the dimerization, endocytosis, degradation and signaling of plant sucrose transporters .
準備方法
a. Synthetic Routes
Sucralose is synthesized from sucrose through a multistep process The key steps involve chlorination and selective dechlorination
Dechlorination: The chlorinated sucrose is then selectively dechlorinated to form sucralose by removing specific chlorine atoms.
b. Industrial Production
The industrial production of sucralose involves large-scale manufacturing processes. These include:
Chlorination: Sucrose is chlorinated using chlorine and acetic anhydride.
Dechlorination: The chlorinated product is treated with methanol and sodium methoxide to remove chlorine atoms.
Purification: The resulting sucralose is purified to remove impurities.
化学反応の分析
スクラロースは非常に安定しており、通常の条件下では容易に化学反応を起こしません。 高温での食品加工中に分解し、潜在的に有害な副生成物が生成される可能性があることに注意することが重要です 。一般的な反応には、加水分解と脱塩素化があります。
4. 科学研究への応用
スクラロースは、さまざまな分野で応用されています。
食品および飲料業界: 飲料、乳製品、ジャム、シロップ、菓子などに使用されます。
健康と医療: 肥満、心臓血管疾患、糖尿病の管理のための低カロリー製品に含まれています。
食品加工: 低温殺菌や噴霧乾燥など、高温プロセスに適しています。
類似化合物との比較
Sucralose stands out due to its origin from sucrose and its high sweetness. Other similar compounds include aspartame, saccharin, and steviol glycosides.
生物活性
Sucralose is a non-caloric artificial sweetener that is approximately 600 times sweeter than sucrose. It is widely used in food and beverage products as a sugar substitute. Despite its popularity, there has been increasing scrutiny regarding its biological effects, particularly concerning metabolic health, immune function, and gut microbiota. This article aims to provide a detailed examination of the biological activity of sucralose, highlighting recent research findings and their implications for health.
Immunomodulatory Effects
Recent studies have demonstrated that high doses of sucralose can negatively impact immune function. Research conducted on mice showed that sucralose intake limited T cell proliferation and differentiation, which are critical for an effective immune response. Specifically, sucralose exposure resulted in decreased CD8+ T cell responses in models of cancer and bacterial infections. The mechanism identified involves alterations in T cell membrane order and impaired T cell receptor signaling, leading to reduced intracellular calcium mobilization and delayed phosphorylation of important signaling proteins like PLCγ1 .
Metabolic Effects
Sucralose has been found to influence various metabolic pathways:
- Insulin Response : Sucralose activates sweet taste receptors in pancreatic β-cells, leading to increased secretion of insulin and incretin hormones such as GLP-1 and GIP. This response may be contingent on direct carbohydrate exposure to the intestinal mucosa .
- Adipogenesis : In vitro studies indicated that sucralose exposure elevated reactive oxygen species (ROS) levels in adipose-derived mesenchymal stem cells, suggesting a potential increase in adipogenesis .
- Gut Microbiota : Sucralose consumption has been associated with dysbiosis—an imbalance in gut microbiota—which may lead to adverse metabolic outcomes. Changes in the composition of gut bacteria can affect liver function and overall metabolism, potentially contributing to conditions like metabolic syndrome .
Absorption, Distribution, Metabolism, and Excretion (ADME)
Sucralose is not fully absorbed in the gastrointestinal tract; approximately 85% is excreted unchanged in urine. However, the small fraction that is absorbed can influence metabolic processes:
- Absorption : Sucralose is rapidly absorbed after ingestion but does not significantly bind to blood proteins, which limits its impact on metabolic pathways .
- Distribution : It distributes throughout the body but primarily affects tissues involved in metabolism and digestion.
- Metabolism : Unlike other sweeteners, sucralose undergoes minimal metabolism; thus, its effects are largely attributed to its interaction with taste receptors rather than direct metabolic conversion .
Case Studies and Research Findings
- Case Study on Immune Function :
- Impact on Gut Microbiota :
- Metabolic Syndrome Implications :
Summary Table of Biological Effects
特性
IUPAC Name |
2-[2,5-bis(chloromethyl)-3,4-dihydroxyoxolan-2-yl]oxy-5-chloro-6-(hydroxymethyl)oxane-3,4-diol | |
---|---|---|
Details | Computed by Lexichem TK 2.7.0 (PubChem release 2021.05.07) | |
Source | PubChem | |
URL | https://pubchem.ncbi.nlm.nih.gov | |
Description | Data deposited in or computed by PubChem | |
InChI |
InChI=1S/C12H19Cl3O8/c13-1-4-7(17)10(20)12(3-14,22-4)23-11-9(19)8(18)6(15)5(2-16)21-11/h4-11,16-20H,1-3H2 | |
Details | Computed by InChI 1.0.6 (PubChem release 2021.05.07) | |
Source | PubChem | |
URL | https://pubchem.ncbi.nlm.nih.gov | |
Description | Data deposited in or computed by PubChem | |
InChI Key |
BAQAVOSOZGMPRM-UHFFFAOYSA-N | |
Details | Computed by InChI 1.0.6 (PubChem release 2021.05.07) | |
Source | PubChem | |
URL | https://pubchem.ncbi.nlm.nih.gov | |
Description | Data deposited in or computed by PubChem | |
Canonical SMILES |
C(C1C(C(C(C(O1)OC2(C(C(C(O2)CCl)O)O)CCl)O)O)Cl)O | |
Details | Computed by OEChem 2.3.0 (PubChem release 2021.05.07) | |
Source | PubChem | |
URL | https://pubchem.ncbi.nlm.nih.gov | |
Description | Data deposited in or computed by PubChem | |
Molecular Formula |
C12H19Cl3O8 | |
Details | Computed by PubChem 2.1 (PubChem release 2021.05.07) | |
Source | PubChem | |
URL | https://pubchem.ncbi.nlm.nih.gov | |
Description | Data deposited in or computed by PubChem | |
Molecular Weight |
397.6 g/mol | |
Details | Computed by PubChem 2.1 (PubChem release 2021.05.07) | |
Source | PubChem | |
URL | https://pubchem.ncbi.nlm.nih.gov | |
Description | Data deposited in or computed by PubChem | |
Physical Description |
Solid | |
Record name | Sucralose | |
Source | Human Metabolome Database (HMDB) | |
URL | http://www.hmdb.ca/metabolites/HMDB0031554 | |
Description | The Human Metabolome Database (HMDB) is a freely available electronic database containing detailed information about small molecule metabolites found in the human body. | |
Explanation | HMDB is offered to the public as a freely available resource. Use and re-distribution of the data, in whole or in part, for commercial purposes requires explicit permission of the authors and explicit acknowledgment of the source material (HMDB) and the original publication (see the HMDB citing page). We ask that users who download significant portions of the database cite the HMDB paper in any resulting publications. | |
CAS No. |
56038-13-2 | |
Record name | Sucralose | |
Source | Human Metabolome Database (HMDB) | |
URL | http://www.hmdb.ca/metabolites/HMDB0031554 | |
Description | The Human Metabolome Database (HMDB) is a freely available electronic database containing detailed information about small molecule metabolites found in the human body. | |
Explanation | HMDB is offered to the public as a freely available resource. Use and re-distribution of the data, in whole or in part, for commercial purposes requires explicit permission of the authors and explicit acknowledgment of the source material (HMDB) and the original publication (see the HMDB citing page). We ask that users who download significant portions of the database cite the HMDB paper in any resulting publications. | |
Melting Point |
130 °C | |
Record name | Sucralose | |
Source | Human Metabolome Database (HMDB) | |
URL | http://www.hmdb.ca/metabolites/HMDB0031554 | |
Description | The Human Metabolome Database (HMDB) is a freely available electronic database containing detailed information about small molecule metabolites found in the human body. | |
Explanation | HMDB is offered to the public as a freely available resource. Use and re-distribution of the data, in whole or in part, for commercial purposes requires explicit permission of the authors and explicit acknowledgment of the source material (HMDB) and the original publication (see the HMDB citing page). We ask that users who download significant portions of the database cite the HMDB paper in any resulting publications. | |
Retrosynthesis Analysis
AI-Powered Synthesis Planning: Our tool employs the Template_relevance Pistachio, Template_relevance Bkms_metabolic, Template_relevance Pistachio_ringbreaker, Template_relevance Reaxys, Template_relevance Reaxys_biocatalysis model, leveraging a vast database of chemical reactions to predict feasible synthetic routes.
One-Step Synthesis Focus: Specifically designed for one-step synthesis, it provides concise and direct routes for your target compounds, streamlining the synthesis process.
Accurate Predictions: Utilizing the extensive PISTACHIO, BKMS_METABOLIC, PISTACHIO_RINGBREAKER, REAXYS, REAXYS_BIOCATALYSIS database, our tool offers high-accuracy predictions, reflecting the latest in chemical research and data.
Strategy Settings
Precursor scoring | Relevance Heuristic |
---|---|
Min. plausibility | 0.01 |
Model | Template_relevance |
Template Set | Pistachio/Bkms_metabolic/Pistachio_ringbreaker/Reaxys/Reaxys_biocatalysis |
Top-N result to add to graph | 6 |
Feasible Synthetic Routes
試験管内研究製品の免責事項と情報
BenchChemで提示されるすべての記事および製品情報は、情報提供を目的としています。BenchChemで購入可能な製品は、生体外研究のために特別に設計されています。生体外研究は、ラテン語の "in glass" に由来し、生物体の外で行われる実験を指します。これらの製品は医薬品または薬として分類されておらず、FDAから任何の医療状態、病気、または疾患の予防、治療、または治癒のために承認されていません。これらの製品を人間または動物に体内に導入する形態は、法律により厳格に禁止されています。これらのガイドラインに従うことは、研究と実験において法的および倫理的な基準の遵守を確実にするために重要です。