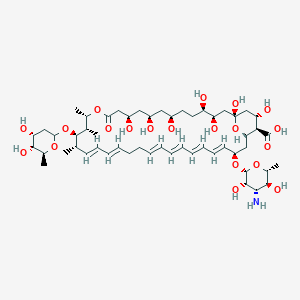
ニスタチンA3
概要
説明
Nystatin A3 is a polyene macrolide antibiotic produced by the bacterium Streptomyces noursei. It is closely related to other nystatin analogues and is known for its potent antifungal properties. Nystatin A3 is particularly effective against Candida species and other fungi, making it a valuable compound in the treatment of fungal infections .
科学的研究の応用
Nystatin A3 has a wide range of scientific research applications, including:
Chemistry: Used as a model compound for studying polyene macrolide antibiotics and their chemical modifications.
Biology: Investigated for its interactions with fungal cell membranes and its role in disrupting fungal cell integrity.
Medicine: Employed in the treatment of fungal infections, particularly those caused by Candida species. It is also studied for its potential use in treating other fungal pathogens.
Industry: Utilized in the development of antifungal formulations and as a preservative in various products.
作用機序
Target of Action
Nystatin A3, like other polyene antifungal drugs, primarily targets ergosterol , a sterol present in the cell membranes of fungi, most notably Candida species . Ergosterol plays a crucial role in maintaining the integrity and fluidity of fungal cell membranes. The interaction between Nystatin A3 and ergosterol is key to its antifungal activity .
Mode of Action
Nystatin A3 exerts its antifungal effects by disrupting the fungal cell membrane. It acts as a channel-forming ionophore , creating pores in the fungal plasma membrane . The formation of these pores changes the membrane’s permeability, allowing for leakage of intracellular contents, which ultimately leads to cell death . This interaction is driven by the presence of highly ordered membrane domains capable of stabilizing the Nystatin oligomers .
Biochemical Pathways
The primary antifungal mechanism of Nystatin involves the formation of transmembrane channels that cause the leakage of cellular K+ and Mg2+, eventually leading to the death of the fungal cells . The interactions between Nystatin molecules and ergosterol seem to occur through the conjugated double bonds of the Nystatin molecules .
Pharmacokinetics
This limits its efficacy to the treatment/prevention of cutaneous, mucocutaneous, and gastrointestinal fungal infections . The compound’s ADME (Absorption, Distribution, Metabolism, and Excretion) properties significantly impact its bioavailability and therapeutic effectiveness.
Result of Action
The result of Nystatin A3’s action at the molecular and cellular level is the disruption of the fungal cell membrane, leading to cell death . This is achieved through the formation of pores in the membrane, which cause leakage of intracellular contents . In addition, Nystatin-induced pore formation is accompanied by strong Nystatin-induced membrane reorganization that depends on membrane lipid composition .
Action Environment
The action of Nystatin A3 is influenced by the biophysical properties and lipid composition of the membrane . For instance, in membranes enriched in a gel-phase forming phospholipid, Nystatin incorporates within the phospholipid-enriched gel domains, where it forms pores able to expand the gel domains . In contrast, in membranes enriched in gel domain forming sphingolipids, Nystatin-induced pore formation occurs through the destabilization of the gel phase .
Safety and Hazards
Nystatin A3, like other nystatins, can cause hypersensitivity reactions including rash, pruritus, and anaphylactoid reactions . It may also cause adverse reproductive effects, based on experimental data . The chief hazard associated with exposure during normal use and handling is the potential for irritation of contaminated skin .
将来の方向性
Nystatin A3 is a potent antifungal drug with significant toxicity in mammalian organisms . In order to develop a non-toxic and more effective Nystatin A3 formulation, its molecular mechanism of action at the cell membrane needs to be better understood . Future research may focus on understanding the complex mechanism of action of Nystatin A3 and developing safer and more effective formulations .
生化学分析
Biochemical Properties
Nystatin A3 interacts with various biomolecules, primarily membrane sterols, to exert its antifungal effects . It forms aqueous pores in the fungal cell membrane, leading to leakage of intracellular contents and disruption of electrochemical gradients . This interaction is driven by the presence of highly ordered membrane domains capable of stabilizing the Nystatin A3 oligomers .
Cellular Effects
Nystatin A3 has significant effects on various types of cells and cellular processes. It influences cell function by disrupting the integrity of the cell membrane, leading to leakage of intracellular contents . This disruption can affect cell signaling pathways, gene expression, and cellular metabolism .
Molecular Mechanism
The mechanism of action of Nystatin A3 is complex and involves several steps at the molecular level. It forms and stabilizes aqueous pores in the fungal cell membrane, which are associated with its cytotoxicity . This pore formation is accompanied by strong Nystatin A3-induced membrane reorganization that depends on membrane lipid composition .
Temporal Effects in Laboratory Settings
The effects of Nystatin A3 over time in laboratory settings have been observed in studies. For instance, a study showed that Nystatin A3 exhibited higher therapeutic efficacy over time in white mice with generalized candidiasis .
Metabolic Pathways
Nystatin A3 is involved in several metabolic pathways. It interacts with enzymes and cofactors, particularly those involved in the synthesis and regulation of membrane sterols
Transport and Distribution
The transport and distribution of Nystatin A3 within cells and tissues are largely dependent on its interaction with membrane sterols . It is believed to incorporate within phospholipid-enriched gel domains, where it forms pores .
Subcellular Localization
The subcellular localization of Nystatin A3 is primarily at the cell membrane, where it interacts with membrane sterols to form pores This localization is crucial for its antifungal activity
準備方法
Synthetic Routes and Reaction Conditions
Nystatin A3 is typically produced through fermentation processes involving Streptomyces noursei. The bacterium is cultured under specific conditions that promote the production of nystatin compounds. The fermentation broth is then subjected to extraction and purification processes to isolate Nystatin A3 .
Industrial Production Methods
Industrial production of Nystatin A3 involves large-scale fermentation using optimized strains of Streptomyces noursei. The fermentation process is carefully controlled to maximize yield and purity. After fermentation, the compound is extracted using organic solvents and purified through various chromatographic techniques .
化学反応の分析
Types of Reactions
Nystatin A3 undergoes several types of chemical reactions, including:
Oxidation: Nystatin A3 can be oxidized under specific conditions, leading to the formation of various oxidized derivatives.
Reduction: Reduction reactions can modify the polyene structure of Nystatin A3, potentially altering its antifungal activity.
Substitution: Substitution reactions involving the hydroxyl groups of Nystatin A3 can lead to the formation of glycosylated derivatives.
Common Reagents and Conditions
Oxidation: Common oxidizing agents include potassium permanganate and hydrogen peroxide.
Reduction: Reducing agents such as sodium borohydride and lithium aluminum hydride are used.
Substitution: Glycosylation reactions often involve glycosyl donors and catalysts under mild conditions.
Major Products Formed
The major products formed from these reactions include various glycosylated and oxidized derivatives of Nystatin A3, which may exhibit different biological activities .
類似化合物との比較
Similar Compounds
Nystatin A1: Another polyene macrolide antibiotic with similar antifungal properties but differing in its sugar moiety.
Amphotericin B: A related polyene macrolide with a broader spectrum of activity but higher toxicity.
Polyfungin B: A compound with better efficacy against certain fungi compared to Nystatin A3.
Uniqueness
Nystatin A3 is unique due to its specific glycosylation pattern, which influences its solubility and biological activity. Its reduced toxicity compared to other polyene macrolides makes it a valuable alternative in antifungal therapy .
特性
IUPAC Name |
33-(4-amino-3,5-dihydroxy-6-methyloxan-2-yl)oxy-17-(4,5-dihydroxy-6-methyloxan-2-yl)oxy-1,3,4,7,9,11,37-heptahydroxy-15,16,18-trimethyl-13-oxo-14,39-dioxabicyclo[33.3.1]nonatriaconta-19,21,25,27,29,31-hexaene-36-carboxylic acid | |
---|---|---|
Details | Computed by Lexichem TK 2.7.0 (PubChem release 2021.05.07) | |
Source | PubChem | |
URL | https://pubchem.ncbi.nlm.nih.gov | |
Description | Data deposited in or computed by PubChem | |
InChI |
InChI=1S/C53H85NO20/c1-29-18-16-14-12-10-8-6-7-9-11-13-15-17-19-37(72-52-49(65)46(54)48(64)33(5)71-52)25-42-45(51(66)67)41(61)28-53(68,74-42)27-40(60)38(58)21-20-34(55)22-35(56)23-36(57)24-43(62)69-31(3)30(2)50(29)73-44-26-39(59)47(63)32(4)70-44/h6-7,9,11-19,29-42,44-50,52,55-61,63-65,68H,8,10,20-28,54H2,1-5H3,(H,66,67) | |
Details | Computed by InChI 1.0.6 (PubChem release 2021.05.07) | |
Source | PubChem | |
URL | https://pubchem.ncbi.nlm.nih.gov | |
Description | Data deposited in or computed by PubChem | |
InChI Key |
IKYMLQOHQLVORI-UHFFFAOYSA-N | |
Details | Computed by InChI 1.0.6 (PubChem release 2021.05.07) | |
Source | PubChem | |
URL | https://pubchem.ncbi.nlm.nih.gov | |
Description | Data deposited in or computed by PubChem | |
Canonical SMILES |
CC1C=CC=CCCC=CC=CC=CC=CC(CC2C(C(CC(O2)(CC(C(CCC(CC(CC(CC(=O)OC(C(C1OC3CC(C(C(O3)C)O)O)C)C)O)O)O)O)O)O)O)C(=O)O)OC4C(C(C(C(O4)C)O)N)O | |
Details | Computed by OEChem 2.3.0 (PubChem release 2021.05.07) | |
Source | PubChem | |
URL | https://pubchem.ncbi.nlm.nih.gov | |
Description | Data deposited in or computed by PubChem | |
Molecular Formula |
C53H85NO20 | |
Details | Computed by PubChem 2.1 (PubChem release 2021.05.07) | |
Source | PubChem | |
URL | https://pubchem.ncbi.nlm.nih.gov | |
Description | Data deposited in or computed by PubChem | |
DSSTOX Substance ID |
DTXSID80212205 | |
Record name | Nystatin A3 | |
Source | EPA DSSTox | |
URL | https://comptox.epa.gov/dashboard/DTXSID80212205 | |
Description | DSSTox provides a high quality public chemistry resource for supporting improved predictive toxicology. | |
Molecular Weight |
1056.2 g/mol | |
Details | Computed by PubChem 2.1 (PubChem release 2021.05.07) | |
Source | PubChem | |
URL | https://pubchem.ncbi.nlm.nih.gov | |
Description | Data deposited in or computed by PubChem | |
CAS No. |
62997-67-5 | |
Record name | Nystatin A3 | |
Source | EPA DSSTox | |
URL | https://comptox.epa.gov/dashboard/DTXSID80212205 | |
Description | DSSTox provides a high quality public chemistry resource for supporting improved predictive toxicology. | |
Q1: Can the glycosylation pattern of Nystatin A3 be modified to generate novel antifungal agents?
A: Yes, research suggests that modifying the glycosylation of polyene macrolides like nystatin A3 holds promise for developing new antifungal drugs []. Increasing the extent of glycosylation through enzymatic or chemical methods could potentially reduce toxicity while maintaining or improving antifungal activity. This approach is particularly relevant for addressing the side effects associated with existing polyene antifungals.
Q2: What are the implications of identifying novel sugar components in polyene macrolides like Nystatin A3?
A: Discovering a novel sugar component like 2,6-dideoxy-L-ribohexopyranose in Nystatin A3 [] broadens our understanding of polyene macrolide biosynthesis and structural diversity. This finding suggests the existence of specialized glycosyltransferases in the producing organism, opening avenues for bioengineering efforts to generate new polyene analogues with tailored sugar moieties for improved pharmacological properties.
試験管内研究製品の免責事項と情報
BenchChemで提示されるすべての記事および製品情報は、情報提供を目的としています。BenchChemで購入可能な製品は、生体外研究のために特別に設計されています。生体外研究は、ラテン語の "in glass" に由来し、生物体の外で行われる実験を指します。これらの製品は医薬品または薬として分類されておらず、FDAから任何の医療状態、病気、または疾患の予防、治療、または治癒のために承認されていません。これらの製品を人間または動物に体内に導入する形態は、法律により厳格に禁止されています。これらのガイドラインに従うことは、研究と実験において法的および倫理的な基準の遵守を確実にするために重要です。