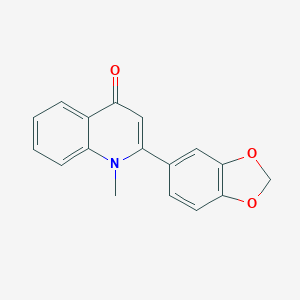
Graveoline
概要
説明
Graveoline is a 2-phenyl quinolinone compound that belongs to the family of alkaloids. This compound exhibits a variety of pharmacological activities, including antifungal, antibacterial, spasmolytic, and anti-tumor properties .
科学的研究の応用
Graveoline has a wide range of scientific research applications:
Chemistry: It is used as a precursor for the synthesis of various quinoline-based compounds.
Medicine: It has shown potential as an acetylcholinesterase inhibitor, which could be useful in the treatment of Alzheimer’s disease.
Industry: The compound’s spasmolytic properties make it useful in the development of muscle relaxants.
作用機序
Target of Action
Graveoline primarily targets cancer cells and fungal cells . In cancer cells, it triggers apoptosis and autophagy, particularly in skin melanoma cells . In fungal cells, specifically Candida albicans, this compound inhibits the growth of the fungus . This compound also exhibits selective inhibitory activity against Acetylcholinesterase (AChE), making it a potential lead compound for the treatment of Alzheimer’s disease .
Mode of Action
this compound interacts with its targets to induce cell death. In cancer cells, it triggers both apoptosis (programmed cell death) and autophagy (self-digestion of cellular components) . These processes are independent events, suggesting that this compound can induce cell death through multiple pathways . In fungal cells, this compound inhibits the growth of Candida albicans at doses of 250 to 500 µg/mL . This compound analogs have also been shown to inhibit AChE, which could potentially slow the progression of Alzheimer’s disease .
Biochemical Pathways
this compound affects several biochemical pathways. In cancer cells, it induces apoptosis and autophagy, leading to cell death . In fungal cells, it targets the Isocitrate lyase (ICL1) gene, a key enzyme in the glyoxylate cycle that contributes to the virulence potential of Candida albicans . The inhibition of this gene leads to the suppression of fungal growth .
Pharmacokinetics
In silico analysis suggests a good prediction of these chemical properties .
Result of Action
The result of this compound’s action is the death of targeted cells. In cancer cells, this compound induces apoptosis and autophagy, leading to cell death . In fungal cells, this compound inhibits the growth of Candida albicans . This compound analogs also inhibit AChE, which could potentially slow the progression of Alzheimer’s disease .
Safety and Hazards
将来の方向性
生化学分析
Biochemical Properties
Graveoline plays a crucial role in various biochemical reactions. It has been shown to interact with several enzymes, proteins, and other biomolecules. One notable interaction is with the isocitrate lyase enzyme, which is a key enzyme in the glyoxylate cycle of Candida albicans . This compound inhibits the activity of isocitrate lyase, thereby disrupting the metabolic adaptation of Candida albicans and contributing to its antifungal potential . Additionally, this compound has been found to interact with other biomolecules, such as proteins involved in cell signaling pathways, further influencing its biochemical properties.
Cellular Effects
This compound exerts significant effects on various types of cells and cellular processes. In Candida albicans, this compound inhibits cell growth by downregulating the expression of the isocitrate lyase gene (ICL1) and its corresponding protein . This inhibition disrupts the glyoxylate cycle, leading to impaired cellular metabolism and reduced virulence of the fungal pathogen . Moreover, this compound has been shown to influence cell signaling pathways, gene expression, and cellular metabolism in other cell types, contributing to its broad-spectrum pharmacological activities.
Molecular Mechanism
The molecular mechanism of this compound involves its binding interactions with biomolecules, enzyme inhibition, and changes in gene expression. This compound binds to the active site of isocitrate lyase, inhibiting its enzymatic activity and disrupting the glyoxylate cycle in Candida albicans . This inhibition leads to a decrease in the production of essential metabolites, ultimately impairing the pathogen’s ability to survive and proliferate . Additionally, this compound’s interaction with other proteins and enzymes further modulates cellular processes, contributing to its overall pharmacological effects.
Temporal Effects in Laboratory Settings
In laboratory settings, the effects of this compound have been observed to change over time. Studies have shown that this compound remains stable under various conditions, maintaining its antifungal activity over extended periods . Prolonged exposure to this compound may lead to its gradual degradation, resulting in reduced efficacy . Long-term studies have also indicated that this compound can have lasting effects on cellular function, including sustained inhibition of isocitrate lyase activity and persistent disruption of cellular metabolism .
Dosage Effects in Animal Models
The effects of this compound vary with different dosages in animal models. At lower doses, this compound exhibits potent antifungal activity without causing significant toxicity . At higher doses, this compound may induce toxic or adverse effects, including hepatotoxicity and nephrotoxicity . Threshold effects have been observed, where the efficacy of this compound reaches a plateau beyond a certain dosage, indicating the importance of optimizing dosage levels for therapeutic applications .
Metabolic Pathways
This compound is involved in several metabolic pathways, including the glyoxylate cycle in Candida albicans . By inhibiting isocitrate lyase, this compound disrupts the production of key metabolites, leading to impaired cellular metabolism and reduced virulence of the fungal pathogen . Additionally, this compound interacts with other enzymes and cofactors, influencing metabolic flux and metabolite levels in various cell types .
Transport and Distribution
This compound is transported and distributed within cells and tissues through specific transporters and binding proteins. These interactions facilitate its localization and accumulation in target cells, enhancing its pharmacological effects . The transport and distribution of this compound are crucial for its efficacy, as they determine the compound’s bioavailability and therapeutic potential .
Subcellular Localization
The subcellular localization of this compound plays a significant role in its activity and function. This compound is directed to specific compartments or organelles within cells through targeting signals and post-translational modifications . This localization enhances its interaction with target biomolecules, such as isocitrate lyase, and contributes to its overall pharmacological effects .
準備方法
Graveoline can be synthesized through several methods. One common synthetic route involves the cyclization of 2-aryl amides with a base to form quinolone derivatives . Another method utilizes quinolin-4-ones as convenient common precursors for a metal-free total synthesis of this compound . The reaction conditions typically involve intramolecular cyclization, oxidation/aromatization, N-methylation, and oxidative C-C bond processes .
化学反応の分析
Graveoline undergoes various chemical reactions, including:
Oxidation: This reaction can be carried out using oxidizing agents such as potassium permanganate or chromium trioxide.
Reduction: Reduction reactions can be performed using reducing agents like sodium borohydride or lithium aluminum hydride.
Substitution: Substitution reactions often involve reagents like halogens or alkylating agents
The major products formed from these reactions depend on the specific reagents and conditions used. For example, oxidation of this compound can lead to the formation of quinolinone derivatives, while reduction can yield amine derivatives .
類似化合物との比較
Graveoline is structurally similar to other alkaloids such as arborinine and dubamine . it is unique in its combination of pharmacological activities, particularly its dual role as an acetylcholinesterase inhibitor and an antifungal agent . This makes it a valuable compound for diverse scientific research and therapeutic applications.
特性
IUPAC Name |
2-(1,3-benzodioxol-5-yl)-1-methylquinolin-4-one | |
---|---|---|
Source | PubChem | |
URL | https://pubchem.ncbi.nlm.nih.gov | |
Description | Data deposited in or computed by PubChem | |
InChI |
InChI=1S/C17H13NO3/c1-18-13-5-3-2-4-12(13)15(19)9-14(18)11-6-7-16-17(8-11)21-10-20-16/h2-9H,10H2,1H3 | |
Source | PubChem | |
URL | https://pubchem.ncbi.nlm.nih.gov | |
Description | Data deposited in or computed by PubChem | |
InChI Key |
COBBNRKBTCBWQP-UHFFFAOYSA-N | |
Source | PubChem | |
URL | https://pubchem.ncbi.nlm.nih.gov | |
Description | Data deposited in or computed by PubChem | |
Canonical SMILES |
CN1C2=CC=CC=C2C(=O)C=C1C3=CC4=C(C=C3)OCO4 | |
Source | PubChem | |
URL | https://pubchem.ncbi.nlm.nih.gov | |
Description | Data deposited in or computed by PubChem | |
Molecular Formula |
C17H13NO3 | |
Source | PubChem | |
URL | https://pubchem.ncbi.nlm.nih.gov | |
Description | Data deposited in or computed by PubChem | |
DSSTOX Substance ID |
DTXSID80326593 | |
Record name | Graveoline | |
Source | EPA DSSTox | |
URL | https://comptox.epa.gov/dashboard/DTXSID80326593 | |
Description | DSSTox provides a high quality public chemistry resource for supporting improved predictive toxicology. | |
Molecular Weight |
279.29 g/mol | |
Source | PubChem | |
URL | https://pubchem.ncbi.nlm.nih.gov | |
Description | Data deposited in or computed by PubChem | |
Physical Description |
Solid | |
Record name | Graveoline | |
Source | Human Metabolome Database (HMDB) | |
URL | http://www.hmdb.ca/metabolites/HMDB0033480 | |
Description | The Human Metabolome Database (HMDB) is a freely available electronic database containing detailed information about small molecule metabolites found in the human body. | |
Explanation | HMDB is offered to the public as a freely available resource. Use and re-distribution of the data, in whole or in part, for commercial purposes requires explicit permission of the authors and explicit acknowledgment of the source material (HMDB) and the original publication (see the HMDB citing page). We ask that users who download significant portions of the database cite the HMDB paper in any resulting publications. | |
CAS No. |
485-61-0 | |
Record name | Graveoline | |
Source | CAS Common Chemistry | |
URL | https://commonchemistry.cas.org/detail?cas_rn=485-61-0 | |
Description | CAS Common Chemistry is an open community resource for accessing chemical information. Nearly 500,000 chemical substances from CAS REGISTRY cover areas of community interest, including common and frequently regulated chemicals, and those relevant to high school and undergraduate chemistry classes. This chemical information, curated by our expert scientists, is provided in alignment with our mission as a division of the American Chemical Society. | |
Explanation | The data from CAS Common Chemistry is provided under a CC-BY-NC 4.0 license, unless otherwise stated. | |
Record name | Graveoline | |
Source | EPA DSSTox | |
URL | https://comptox.epa.gov/dashboard/DTXSID80326593 | |
Description | DSSTox provides a high quality public chemistry resource for supporting improved predictive toxicology. | |
Record name | Graveoline | |
Source | Human Metabolome Database (HMDB) | |
URL | http://www.hmdb.ca/metabolites/HMDB0033480 | |
Description | The Human Metabolome Database (HMDB) is a freely available electronic database containing detailed information about small molecule metabolites found in the human body. | |
Explanation | HMDB is offered to the public as a freely available resource. Use and re-distribution of the data, in whole or in part, for commercial purposes requires explicit permission of the authors and explicit acknowledgment of the source material (HMDB) and the original publication (see the HMDB citing page). We ask that users who download significant portions of the database cite the HMDB paper in any resulting publications. | |
Melting Point |
205 - 205.5 °C | |
Record name | Graveoline | |
Source | Human Metabolome Database (HMDB) | |
URL | http://www.hmdb.ca/metabolites/HMDB0033480 | |
Description | The Human Metabolome Database (HMDB) is a freely available electronic database containing detailed information about small molecule metabolites found in the human body. | |
Explanation | HMDB is offered to the public as a freely available resource. Use and re-distribution of the data, in whole or in part, for commercial purposes requires explicit permission of the authors and explicit acknowledgment of the source material (HMDB) and the original publication (see the HMDB citing page). We ask that users who download significant portions of the database cite the HMDB paper in any resulting publications. | |
Retrosynthesis Analysis
AI-Powered Synthesis Planning: Our tool employs the Template_relevance Pistachio, Template_relevance Bkms_metabolic, Template_relevance Pistachio_ringbreaker, Template_relevance Reaxys, Template_relevance Reaxys_biocatalysis model, leveraging a vast database of chemical reactions to predict feasible synthetic routes.
One-Step Synthesis Focus: Specifically designed for one-step synthesis, it provides concise and direct routes for your target compounds, streamlining the synthesis process.
Accurate Predictions: Utilizing the extensive PISTACHIO, BKMS_METABOLIC, PISTACHIO_RINGBREAKER, REAXYS, REAXYS_BIOCATALYSIS database, our tool offers high-accuracy predictions, reflecting the latest in chemical research and data.
Strategy Settings
Precursor scoring | Relevance Heuristic |
---|---|
Min. plausibility | 0.01 |
Model | Template_relevance |
Template Set | Pistachio/Bkms_metabolic/Pistachio_ringbreaker/Reaxys/Reaxys_biocatalysis |
Top-N result to add to graph | 6 |
Feasible Synthetic Routes
A: Graveoline triggers both apoptosis and autophagy in cancer cells. [] It induces Beclin-1 associated autophagy, an independent process from apoptosis. [] This dual action makes it a promising candidate for combating apoptosis-resistant cancer cells. [] Additionally, this compound induces the generation of reactive oxygen species (ROS), which contribute to autophagy. []
A: No, using 3-methyladenine, an autophagy inhibitor, did not affect apoptosis induced by this compound in skin melanoma cells. This suggests that this compound triggers apoptosis and autophagy via distinct pathways. []
A: Similar to the previous answer, using a caspase inhibitor to block apoptosis did not affect autophagic cell death induced by this compound. This further supports the finding that this compound triggers these two cell death mechanisms independently. []
A: Blocking autophagy did not affect ROS generation induced by this compound, suggesting that ROS generation occurs upstream of autophagy in the this compound-induced cell death pathway. []
A: Yes, this compound exhibits herbicidal activity by acting as a photosynthesis inhibitor, particularly targeting electron transport during the light-dependent reactions. []
A: this compound has a molecular formula of C17H13NO3 and a molecular weight of 279.29 g/mol. [, ]
A: this compound's structure has been confirmed using various spectroscopic methods, primarily 1D and 2D NMR analyses, including 1H NMR, 13C NMR, COSY, HSQC, and HMBC. []
A: Yes, this compound was identified as a potential anaplastic lymphoma kinase (ALK) inhibitor through virtual screening of a traditional Chinese medicine database. [] Molecular docking studies were employed to assess its binding affinity to ALK. []
A: Yes, an in silico study utilized pharmacology networking and molecular docking to investigate this compound's potential against Alzheimer's disease. [] This study suggests that this compound could impact AD pathogenesis through interactions with various targets, including the serotonergic synapse signaling pathway, hormones, anti-neuroinflammatory pathways, transcription regulation, and molecular chaperones. []
A: Studies on this compound analogs revealed that a six-carbon linker (n = 3) between the phenyl and amino terminal groups of the this compound scaffold, with piperidine as the terminal amino group, resulted in the most potent AChE inhibition. [] This modification also led to increased selectivity for AChE over butyrylcholinesterase (BuChE). []
A: In vitro studies using rat and human liver microsomes and hepatocytes revealed that this compound undergoes extensive phase I and phase II metabolism. [] Identified metabolic pathways include demethylenation, demethylation, hydroxylation, glucuronidation, and glutathione conjugation. [] The major metabolite, M10, is formed by opening the methylenedioxyphenyl ring and is primarily catalyzed by CYP1A2, 2C8, 2C9, 2C19, 2D6, 3A4, and 3A5 enzymes. []
A: this compound has demonstrated cytotoxic activity against various human cancer cell lines in vitro, including skin melanoma (A375), [] KB, Hela, DLD, NCI, and Hepa. []
ANone: While this compound has shown promising in vitro anticancer activity, the provided research papers do not provide information on in vivo studies or clinical trials.
ANone: While the provided research papers highlight the potential therapeutic benefits of this compound, it's crucial to acknowledge that Ruta graveolens , the plant from which this compound is extracted, is known to be toxic at higher doses. More research is needed to determine the specific toxicity profile of this compound.
ANone: Given its potent biological activities, careful consideration of this compound's safety profile is crucial. Further research is necessary to establish safe dosages and evaluate potential long-term effects.
A: this compound is usually isolated from plant material using bioassay-guided fractionation techniques. [, , , ] This involves testing different fractions of the plant extract for their desired biological activity, followed by further purification of the active fractions. [, , , ]
A: this compound can be quantified using chromatographic techniques like High-Performance Liquid Chromatography (HPLC). [] Coupling HPLC with detectors such as photodiode array detectors (PDA) or mass spectrometry (MS) allows for sensitive detection and quantification of this compound in complex mixtures. []
A: this compound was first isolated from Ruta graveolens in 1964 by Arthur, Johns, and Lamberton. [] They characterized it as a novel alkaloid and proposed its structure as a 1-methyl-methylenedioxyphenyl-4-quinolone. []
試験管内研究製品の免責事項と情報
BenchChemで提示されるすべての記事および製品情報は、情報提供を目的としています。BenchChemで購入可能な製品は、生体外研究のために特別に設計されています。生体外研究は、ラテン語の "in glass" に由来し、生物体の外で行われる実験を指します。これらの製品は医薬品または薬として分類されておらず、FDAから任何の医療状態、病気、または疾患の予防、治療、または治癒のために承認されていません。これらの製品を人間または動物に体内に導入する形態は、法律により厳格に禁止されています。これらのガイドラインに従うことは、研究と実験において法的および倫理的な基準の遵守を確実にするために重要です。