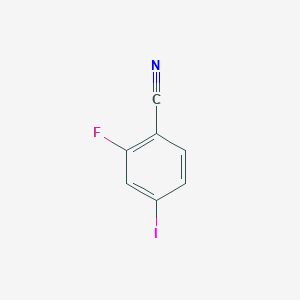
2-Fluoro-4-iodobenzonitrile
概要
説明
2-Fluoro-4-iodo benzonitrile is a building block. It has been used in the synthesis of L. infantum trypanothione reductase (Li-TryR) dimerization and oxidoreductase activity inhibitors. 2-Fluoro-4-iodo benzonitrile has also been used in the synthesis of transient receptor potential ankyrin 1 (TRPA1) antagonists.
生物活性
2-Fluoro-4-iodobenzonitrile (CAS No. 137553-42-5) is a fluorinated aromatic compound that has garnered attention in medicinal chemistry for its potential biological activities. This article aims to provide a comprehensive overview of its biological activity, including its synthesis, mechanisms of action, and relevant case studies.
This compound is characterized by the following chemical properties:
Property | Value |
---|---|
Molecular Formula | CHFIN |
Molecular Weight | 247.01 g/mol |
Boiling Point | Not specified |
Log P (octanol-water) | 1.92 |
TPSA (Topological Polar Surface Area) | 23.79 Ų |
The presence of both fluorine and iodine atoms contributes to its unique reactivity and biological interactions.
Synthesis
The synthesis of this compound typically involves halogenation reactions, where the introduction of fluorine and iodine is achieved through electrophilic aromatic substitution or direct halogenation methods. This compound serves as a versatile building block in organic synthesis, particularly in the development of pharmaceuticals.
This compound has been identified as an inhibitor of various biological targets, including:
- Leishmania infantum Trypanothione Reductase (Li-TryR) : It has been utilized in the synthesis of inhibitors targeting Li-TryR, which is crucial for the survival of the parasite causing leishmaniasis. The inhibition of this enzyme disrupts the redox balance within the parasite, leading to its death .
- Transient Receptor Potential Ankyrin 1 (TRPA1) : This compound has also been explored as a potential antagonist for TRPA1, a receptor involved in pain sensation and inflammatory responses. TRPA1 antagonists may provide new avenues for pain management therapies .
- Antimutagenic Activity : In studies evaluating its antimutagenic properties, this compound exhibited significant activity against mutagens such as sodium azide and benzo[a]pyrene. This suggests that it may play a role in DNA repair mechanisms and cancer prevention .
Case Studies
Several studies have highlighted the biological efficacy of this compound:
- Inhibition Studies : Research demonstrated that derivatives of this compound showed potent inhibition against various kinases involved in cancer pathways. For instance, compounds based on its structure displayed IC values in the nanomolar range against BCR-ABL mutants associated with chronic myeloid leukemia (CML) .
- Anticancer Research : A study focused on fluoroaryl derivatives indicated that modifications to the benzene ring could enhance anticancer activity. The introduction of fluorine increased lipophilicity, improving bioavailability and efficacy against cancer cell lines .
- Ames Test : In mutagenicity assays using Salmonella typhimurium, this compound demonstrated reduced mutagenicity compared to non-fluorinated analogs, highlighting its potential as a safer alternative in drug development .
科学的研究の応用
Pharmaceutical Intermediates
2-Fluoro-4-iodobenzonitrile is primarily utilized as an intermediate in pharmaceutical synthesis. Its unique functional groups allow it to participate in various chemical reactions essential for drug development. The compound's reactivity facilitates the formation of more complex structures, which are crucial in the design of novel therapeutic agents .
Organic Synthesis
This compound serves as a building block in organic synthesis, particularly in constructing heterocyclic compounds and other complex organic molecules. Its ability to undergo nucleophilic substitution reactions makes it valuable for synthesizing derivatives that can be further modified for specific applications .
Biochemical Assays
In biological research, this compound has been employed as a probe in biochemical assays to study enzyme interactions. Its fluorine and iodine substituents can influence molecular recognition and binding affinity, making it useful for investigating enzyme kinetics and mechanisms .
Material Science
The compound is also explored in material science for developing specialty chemicals and materials with specific properties. Its unique structure may contribute to enhanced performance characteristics in various applications, including electronic materials and coatings .
Case Study 1: Synthesis of Antiviral Agents
Research has highlighted the use of this compound in synthesizing antiviral agents. The compound's reactivity allows it to form key intermediates that are essential for developing drugs targeting viral infections. Studies have shown that modifications to the benzonitrile structure can lead to increased efficacy against specific viral strains .
Case Study 2: Investigation of Enzyme Inhibitors
Another study focused on using this compound to develop enzyme inhibitors that could potentially treat metabolic disorders. By altering the substituents on the benzene ring, researchers were able to enhance the binding affinity of the inhibitors towards their targets, demonstrating the compound's utility in drug design .
Q & A
Basic Questions
Q. What are the optimal synthetic routes for preparing 2-fluoro-4-iodobenzonitrile?
A common method involves iodination of 2-fluoro-4-bromobenzonitrile via halogen exchange. This can be achieved using sodium iodide (NaI) in a polar aprotic solvent like dimethylformamide (DMF) or acetonitrile, often under reflux conditions (80–100°C) for 12–24 hours . Alternatively, direct electrophilic iodination of 2-fluorobenzonitrile derivatives using iodine monochloride (ICl) or N-iodosuccinimide (NIS) in the presence of a Lewis acid catalyst (e.g., FeCl₃) may be employed. Post-synthesis purification typically involves column chromatography (silica gel, hexane/ethyl acetate gradient) or recrystallization from ethanol .
Q. Which spectroscopic techniques are most effective for characterizing this compound?
- NMR Spectroscopy : NMR is critical for confirming the fluorine environment (δ ≈ -110 ppm for aromatic F). NMR can identify aromatic protons adjacent to electron-withdrawing groups (e.g., δ 7.5–8.5 ppm for deshielded protons) .
- Mass Spectrometry : High-resolution mass spectrometry (HRMS) confirms the molecular ion peak (expected m/z ≈ 261.92 for C₇H₃FNI⁺).
- IR Spectroscopy : A strong nitrile stretch (~2220 cm⁻¹) and C-F vibrations (~1250 cm⁻¹) are key identifiers .
Q. How should this compound be stored to ensure stability?
Store the compound in amber vials under inert atmosphere (argon or nitrogen) at 2–8°C. The iodine substituent is light-sensitive and prone to hydrolysis; thus, moisture-free conditions are essential. Stability tests indicate <5% degradation over 6 months when stored properly .
Q. What are the primary research applications of this compound?
It serves as a versatile intermediate in cross-coupling reactions (e.g., Suzuki-Miyaura coupling with boronic acids to form biaryl systems) and radiolabeling studies (iodine-125/131 for tracer development). Its electron-deficient aromatic ring also makes it useful in metal-organic framework (MOF) synthesis .
Advanced Research Questions
Q. How does the iodine substituent influence reactivity in palladium-catalyzed cross-coupling reactions?
The C-I bond’s moderate strength (≈50 kcal/mol) facilitates oxidative addition with Pd(0) catalysts (e.g., Pd(PPh₃)₄), enabling efficient coupling with boronic acids or alkynes. However, competing homocoupling or protodeiodination can occur if reaction conditions (temperature, base, solvent) are suboptimal. Using ligand-accelerated catalysis (e.g., XPhos) or microwave-assisted heating (100–120°C) improves yield and selectivity .
Q. How can computational chemistry aid in predicting substitution patterns in derivatives of this compound?
Density functional theory (DFT) calculations (e.g., B3LYP/6-31G*) can model electronic effects of substituents. For example, introducing electron-donating groups (e.g., -NH₂) at the 3-position increases electron density at the nitrile carbon, altering reactivity in nucleophilic additions. Hirshfeld surface analysis further visualizes intermolecular interactions (e.g., halogen bonding with iodine) .
Q. How should researchers address contradictions in reported synthetic yields for this compound derivatives?
Discrepancies often arise from impurities in starting materials or incomplete halogen exchange . Systematic analysis includes:
- Kinetic monitoring : Use TLC or in-situ IR to track reaction progress.
- Isotopic labeling : Introduce or to distinguish desired product from byproducts.
- Statistical optimization : Apply design of experiments (DoE) to variables like temperature, stoichiometry, and catalyst loading .
Q. What strategies are effective for designing bioactive derivatives of this compound?
- Bioisosteric replacement : Substitute iodine with trifluoromethyl (-CF₃) to enhance metabolic stability while retaining steric bulk.
- Prodrug development : Convert the nitrile to a tetrazole moiety for improved solubility and target engagement.
- Structure-activity relationship (SAR) studies : Vary substituents at the 5-position to modulate binding affinity in kinase inhibitors .
Q. What methods resolve purification challenges for this compound in scaled-up syntheses?
- High-performance liquid chromatography (HPLC) : Use C18 columns with acetonitrile/water gradients for high-purity isolation (>99%).
- Crystallization optimization : Screen solvents (e.g., ethanol/toluene mixtures) to improve crystal habit and reduce occluded impurities.
- Byproduct identification : Employ LC-MS to detect and quantify side products (e.g., dehalogenated species) .
特性
IUPAC Name |
2-fluoro-4-iodobenzonitrile | |
---|---|---|
Source | PubChem | |
URL | https://pubchem.ncbi.nlm.nih.gov | |
Description | Data deposited in or computed by PubChem | |
InChI |
InChI=1S/C7H3FIN/c8-7-3-6(9)2-1-5(7)4-10/h1-3H | |
Source | PubChem | |
URL | https://pubchem.ncbi.nlm.nih.gov | |
Description | Data deposited in or computed by PubChem | |
InChI Key |
WHQVXHBSTRFRCE-UHFFFAOYSA-N | |
Source | PubChem | |
URL | https://pubchem.ncbi.nlm.nih.gov | |
Description | Data deposited in or computed by PubChem | |
Canonical SMILES |
C1=CC(=C(C=C1I)F)C#N | |
Source | PubChem | |
URL | https://pubchem.ncbi.nlm.nih.gov | |
Description | Data deposited in or computed by PubChem | |
Molecular Formula |
C7H3FIN | |
Source | PubChem | |
URL | https://pubchem.ncbi.nlm.nih.gov | |
Description | Data deposited in or computed by PubChem | |
DSSTOX Substance ID |
DTXSID80379069 | |
Record name | 2-fluoro-4-iodobenzonitrile | |
Source | EPA DSSTox | |
URL | https://comptox.epa.gov/dashboard/DTXSID80379069 | |
Description | DSSTox provides a high quality public chemistry resource for supporting improved predictive toxicology. | |
Molecular Weight |
247.01 g/mol | |
Source | PubChem | |
URL | https://pubchem.ncbi.nlm.nih.gov | |
Description | Data deposited in or computed by PubChem | |
CAS No. |
137553-42-5 | |
Record name | 2-Fluoro-4-iodobenzonitrile | |
Source | CAS Common Chemistry | |
URL | https://commonchemistry.cas.org/detail?cas_rn=137553-42-5 | |
Description | CAS Common Chemistry is an open community resource for accessing chemical information. Nearly 500,000 chemical substances from CAS REGISTRY cover areas of community interest, including common and frequently regulated chemicals, and those relevant to high school and undergraduate chemistry classes. This chemical information, curated by our expert scientists, is provided in alignment with our mission as a division of the American Chemical Society. | |
Explanation | The data from CAS Common Chemistry is provided under a CC-BY-NC 4.0 license, unless otherwise stated. | |
Record name | 2-fluoro-4-iodobenzonitrile | |
Source | EPA DSSTox | |
URL | https://comptox.epa.gov/dashboard/DTXSID80379069 | |
Description | DSSTox provides a high quality public chemistry resource for supporting improved predictive toxicology. | |
Synthesis routes and methods
Procedure details
Retrosynthesis Analysis
AI-Powered Synthesis Planning: Our tool employs the Template_relevance Pistachio, Template_relevance Bkms_metabolic, Template_relevance Pistachio_ringbreaker, Template_relevance Reaxys, Template_relevance Reaxys_biocatalysis model, leveraging a vast database of chemical reactions to predict feasible synthetic routes.
One-Step Synthesis Focus: Specifically designed for one-step synthesis, it provides concise and direct routes for your target compounds, streamlining the synthesis process.
Accurate Predictions: Utilizing the extensive PISTACHIO, BKMS_METABOLIC, PISTACHIO_RINGBREAKER, REAXYS, REAXYS_BIOCATALYSIS database, our tool offers high-accuracy predictions, reflecting the latest in chemical research and data.
Strategy Settings
Precursor scoring | Relevance Heuristic |
---|---|
Min. plausibility | 0.01 |
Model | Template_relevance |
Template Set | Pistachio/Bkms_metabolic/Pistachio_ringbreaker/Reaxys/Reaxys_biocatalysis |
Top-N result to add to graph | 6 |
Feasible Synthetic Routes
試験管内研究製品の免責事項と情報
BenchChemで提示されるすべての記事および製品情報は、情報提供を目的としています。BenchChemで購入可能な製品は、生体外研究のために特別に設計されています。生体外研究は、ラテン語の "in glass" に由来し、生物体の外で行われる実験を指します。これらの製品は医薬品または薬として分類されておらず、FDAから任何の医療状態、病気、または疾患の予防、治療、または治癒のために承認されていません。これらの製品を人間または動物に体内に導入する形態は、法律により厳格に禁止されています。これらのガイドラインに従うことは、研究と実験において法的および倫理的な基準の遵守を確実にするために重要です。