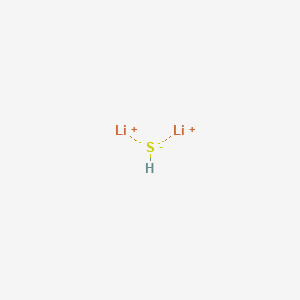
Lithium sulphide, anhydrous
- Cliquez sur DEMANDE RAPIDE pour recevoir un devis de notre équipe d'experts.
- Avec des produits de qualité à un prix COMPÉTITIF, vous pouvez vous concentrer davantage sur votre recherche.
Vue d'ensemble
Description
Lithium sulphide, anhydrous, is an inorganic compound with the chemical formula Li₂S. It appears as a solid yellow-white deliquescent powder and is known for its high reactivity with water, releasing hydrogen sulphide gas upon hydrolysis . This compound crystallizes in the antifluorite motif and is described as the salt (Li⁺)₂S²⁻ .
Méthodes De Préparation
Synthetic Routes and Reaction Conditions:
-
Direct Synthesis: Lithium sulphide can be synthesized by directly reacting lithium with sulphur in anhydrous ammonia. The reaction is as follows: [ 2 \text{Li} + \text{S} \rightarrow \text{Li}_2\text{S} ] This method is convenient and straightforward .
-
Metathesis Reactions: Another method involves metathesis reactions between lithium salts (such as lithium halides or nitrates) and sodium sulphide. This method is economical, scalable, and environmentally friendly .
-
Reduction of Lithium Sulphate: Lithium sulphide can also be prepared by reducing lithium sulphate with hydrogen. This green and cost-effective method avoids side reactions and produces high-purity lithium sulphide .
Analyse Des Réactions Chimiques
Types of Reactions:
Oxidation: Lithium sulphide can undergo oxidation reactions, forming lithium polysulphides and eventually lithium sulphate.
Reduction: It can be reduced to lithium and elemental sulphur under specific conditions.
Hydrolysis: In the presence of water, lithium sulphide hydrolyzes to form lithium hydroxide and hydrogen sulphide gas.
Common Reagents and Conditions:
Oxidation: Oxygen or other oxidizing agents.
Reduction: Reducing agents such as hydrogen or lithium triethylborohydride.
Hydrolysis: Water or moisture in the air.
Major Products:
Oxidation: Lithium polysulphides, lithium sulphate.
Reduction: Lithium, elemental sulphur.
Hydrolysis: Lithium hydroxide, hydrogen sulphide gas.
Applications De Recherche Scientifique
Lithium sulphide has several scientific research applications, particularly in the field of energy storage:
Mécanisme D'action
The mechanism of action of lithium sulphide in lithium-sulphur batteries involves the electrochemical reduction and oxidation of sulphur species. During discharge, lithium sulphide is oxidized to form lithium polysulphides, which further oxidize to elemental sulphur. During charging, the reverse reactions occur, reducing sulphur back to lithium sulphide . This redox process is facilitated by the high ionic conductivity of lithium sulphide and its ability to form stable solid-electrolyte interphases .
Comparaison Avec Des Composés Similaires
- Lithium oxide (Li₂O)
- Lithium selenide (Li₂Se)
- Lithium telluride (Li₂Te)
- Lithium polonide (Li₂Po)
- Sodium sulphide (Na₂S)
- Potassium sulphide (K₂S)
- Rubidium sulphide (Rb₂S)
- Caesium sulphide (Cs₂S)
Comparison:
- Lithium oxide (Li₂O): Unlike lithium sulphide, lithium oxide is less reactive with water and does not release hydrogen sulphide gas upon hydrolysis .
- Lithium selenide (Li₂Se) and Lithium telluride (Li₂Te): These compounds have similar structures to lithium sulphide but contain selenium and tellurium, respectively. They exhibit different electrochemical properties and reactivities .
- Sodium sulphide (Na₂S): Sodium sulphide is more soluble in water compared to lithium sulphide and is used in different industrial applications .
Lithium sulphide stands out due to its unique combination of high ionic conductivity, reactivity, and suitability for advanced battery technologies .
Propriétés
Formule moléculaire |
HLi2S+ |
---|---|
Poids moléculaire |
47.0 g/mol |
Nom IUPAC |
dilithium;sulfanide |
InChI |
InChI=1S/2Li.H2S/h;;1H2/q2*+1;/p-1 |
Clé InChI |
ZEBXXUVDYRCDCF-UHFFFAOYSA-M |
SMILES canonique |
[Li+].[Li+].[SH-] |
Description physique |
Yellow powder with an odor of rotten eggs; [MSDSonline] |
Origine du produit |
United States |
Avertissement et informations sur les produits de recherche in vitro
Veuillez noter que tous les articles et informations sur les produits présentés sur BenchChem sont destinés uniquement à des fins informatives. Les produits disponibles à l'achat sur BenchChem sont spécifiquement conçus pour des études in vitro, qui sont réalisées en dehors des organismes vivants. Les études in vitro, dérivées du terme latin "in verre", impliquent des expériences réalisées dans des environnements de laboratoire contrôlés à l'aide de cellules ou de tissus. Il est important de noter que ces produits ne sont pas classés comme médicaments et n'ont pas reçu l'approbation de la FDA pour la prévention, le traitement ou la guérison de toute condition médicale, affection ou maladie. Nous devons souligner que toute forme d'introduction corporelle de ces produits chez les humains ou les animaux est strictement interdite par la loi. Il est essentiel de respecter ces directives pour assurer la conformité aux normes légales et éthiques en matière de recherche et d'expérimentation.