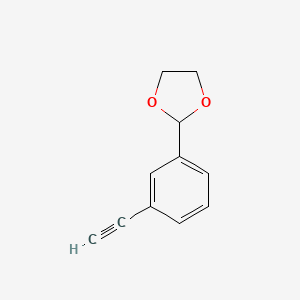
2-(3-Ethynylphenyl)-1,3-dioxolane
- Cliquez sur DEMANDE RAPIDE pour recevoir un devis de notre équipe d'experts.
- Avec des produits de qualité à un prix COMPÉTITIF, vous pouvez vous concentrer davantage sur votre recherche.
Vue d'ensemble
Description
2-(3-Ethynylphenyl)-1,3-dioxolane is a useful research compound. Its molecular formula is C11H10O2 and its molecular weight is 174.20 g/mol. The purity is usually 95%.
BenchChem offers high-quality this compound suitable for many research applications. Different packaging options are available to accommodate customers' requirements. Please inquire for more information about this compound including the price, delivery time, and more detailed information at info@benchchem.com.
Propriétés
Formule moléculaire |
C11H10O2 |
---|---|
Poids moléculaire |
174.20 g/mol |
Nom IUPAC |
2-(3-ethynylphenyl)-1,3-dioxolane |
InChI |
InChI=1S/C11H10O2/c1-2-9-4-3-5-10(8-9)11-12-6-7-13-11/h1,3-5,8,11H,6-7H2 |
Clé InChI |
PJQOKBVNXBFHQW-UHFFFAOYSA-N |
SMILES canonique |
C#CC1=CC(=CC=C1)C2OCCO2 |
Origine du produit |
United States |
Avertissement et informations sur les produits de recherche in vitro
Veuillez noter que tous les articles et informations sur les produits présentés sur BenchChem sont destinés uniquement à des fins informatives. Les produits disponibles à l'achat sur BenchChem sont spécifiquement conçus pour des études in vitro, qui sont réalisées en dehors des organismes vivants. Les études in vitro, dérivées du terme latin "in verre", impliquent des expériences réalisées dans des environnements de laboratoire contrôlés à l'aide de cellules ou de tissus. Il est important de noter que ces produits ne sont pas classés comme médicaments et n'ont pas reçu l'approbation de la FDA pour la prévention, le traitement ou la guérison de toute condition médicale, affection ou maladie. Nous devons souligner que toute forme d'introduction corporelle de ces produits chez les humains ou les animaux est strictement interdite par la loi. Il est essentiel de respecter ces directives pour assurer la conformité aux normes légales et éthiques en matière de recherche et d'expérimentation.