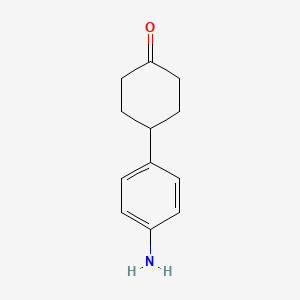
4-(4-aminophenyl)cyclohexan-1-one
- Cliquez sur DEMANDE RAPIDE pour recevoir un devis de notre équipe d'experts.
- Avec des produits de qualité à un prix COMPÉTITIF, vous pouvez vous concentrer davantage sur votre recherche.
Vue d'ensemble
Description
4-(4-aminophenyl)cyclohexan-1-one is an organic compound characterized by the presence of an amino group attached to a phenyl ring, which is further connected to a cyclohexanone moiety
Méthodes De Préparation
Synthetic Routes and Reaction Conditions
The synthesis of 4-(4-aminophenyl)cyclohexan-1-one typically involves the following steps:
Nitration of Phenylcyclohexanone: The starting material, phenylcyclohexanone, undergoes nitration to introduce a nitro group at the para position of the phenyl ring.
Reduction of Nitro Group: The nitro group is then reduced to an amino group using reducing agents such as iron powder or catalytic hydrogenation.
Industrial Production Methods
In an industrial setting, the production of this compound can be scaled up by optimizing reaction conditions such as temperature, pressure, and the use of continuous flow reactors to ensure high yield and purity.
Analyse Des Réactions Chimiques
Types of Reactions
4-(4-aminophenyl)cyclohexan-1-one can undergo various chemical reactions, including:
Oxidation: The amino group can be oxidized to form nitroso or nitro derivatives.
Reduction: The carbonyl group in the cyclohexanone moiety can be reduced to form alcohols.
Substitution: The amino group can participate in nucleophilic substitution reactions to form derivatives with different functional groups.
Common Reagents and Conditions
Oxidation: Common oxidizing agents include potassium permanganate and hydrogen peroxide.
Reduction: Reducing agents such as sodium borohydride or lithium aluminum hydride are used.
Substitution: Reagents like alkyl halides or acyl chlorides can be used for substitution reactions.
Major Products Formed
Oxidation: Formation of nitroso or nitro derivatives.
Reduction: Formation of cyclohexanol derivatives.
Substitution: Formation of N-substituted derivatives.
Applications De Recherche Scientifique
4-(4-aminophenyl)cyclohexan-1-one has several applications in scientific research:
Chemistry: Used as an intermediate in the synthesis of complex organic molecules.
Biology: Studied for its potential biological activity and interactions with biomolecules.
Medicine: Investigated for its potential therapeutic properties, including antimicrobial and anticancer activities.
Industry: Utilized in the production of dyes, pigments, and other industrial chemicals.
Mécanisme D'action
The mechanism of action of 4-(4-aminophenyl)cyclohexan-1-one involves its interaction with specific molecular targets and pathways:
Molecular Targets: The compound can interact with enzymes, receptors, and other proteins, potentially inhibiting or activating their functions.
Pathways Involved: It may influence signaling pathways related to cell growth, apoptosis, and metabolism, leading to its observed biological effects.
Comparaison Avec Des Composés Similaires
Similar Compounds
4-Aminophenol: Similar structure but lacks the cyclohexanone moiety.
4-Aminoacetophenone: Contains a phenyl ring with an amino group and an acetyl group instead of a cyclohexanone moiety.
Uniqueness
4-(4-aminophenyl)cyclohexan-1-one is unique due to the presence of both an amino group and a cyclohexanone moiety, which imparts distinct chemical and biological properties compared to its analogs.
Propriétés
Formule moléculaire |
C12H15NO |
---|---|
Poids moléculaire |
189.25 g/mol |
Nom IUPAC |
4-(4-aminophenyl)cyclohexan-1-one |
InChI |
InChI=1S/C12H15NO/c13-11-5-1-9(2-6-11)10-3-7-12(14)8-4-10/h1-2,5-6,10H,3-4,7-8,13H2 |
Clé InChI |
VKGJREVQGXLTIY-UHFFFAOYSA-N |
SMILES canonique |
C1CC(=O)CCC1C2=CC=C(C=C2)N |
Origine du produit |
United States |
Synthesis routes and methods
Procedure details
Avertissement et informations sur les produits de recherche in vitro
Veuillez noter que tous les articles et informations sur les produits présentés sur BenchChem sont destinés uniquement à des fins informatives. Les produits disponibles à l'achat sur BenchChem sont spécifiquement conçus pour des études in vitro, qui sont réalisées en dehors des organismes vivants. Les études in vitro, dérivées du terme latin "in verre", impliquent des expériences réalisées dans des environnements de laboratoire contrôlés à l'aide de cellules ou de tissus. Il est important de noter que ces produits ne sont pas classés comme médicaments et n'ont pas reçu l'approbation de la FDA pour la prévention, le traitement ou la guérison de toute condition médicale, affection ou maladie. Nous devons souligner que toute forme d'introduction corporelle de ces produits chez les humains ou les animaux est strictement interdite par la loi. Il est essentiel de respecter ces directives pour assurer la conformité aux normes légales et éthiques en matière de recherche et d'expérimentation.