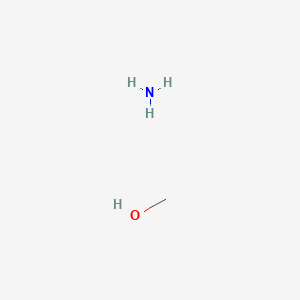
Ammonia methanol
Vue d'ensemble
Description
Ammonia methanol is a useful research compound. Its molecular formula is CH7NO and its molecular weight is 49.073 g/mol. The purity is usually 95%.
BenchChem offers high-quality Ammonia methanol suitable for many research applications. Different packaging options are available to accommodate customers' requirements. Please inquire for more information about Ammonia methanol including the price, delivery time, and more detailed information at info@benchchem.com.
Applications De Recherche Scientifique
Methanol Production from Methane : Ammonia-oxidizing bacteria can convert methane into methanol, offering a high-rate, high-yield process. This has potential applications as a commodity chemical or in nitrogen removal processes (Taher & Chandran, 2013).
Ammonia Degradation : Methanol, used as an auxiliary fuel in supercritical water, promotes ammonia degradation. A remarkable 99.24% removal efficiency was observed at 540°C, making it a potent method for ammonia treatment (J. Zhang et al., 2021).
Fuel Cells : Ammonia is considered a more suitable fuel than methanol in some hydrogen fuel cell applications, attributed to its specific energy and environmental considerations (Metkemeijer & Achard, 1994).
Supercritical Water Oxidation : In the oxidation of ammonia in supercritical water, methanol serves as a more efficient auxiliary fuel compared to isopropanol, achieving nearly complete conversion (Jie Zhang et al., 2019).
Selective Methanol Production : The electrochemical reduction of bicarbonate ions in aqueous ammonia solutions leads to the selective production of methanol, with ammonia playing a key role as an electrolyte (Jiwanti et al., 2016).
Methanol in Ammonia Oxidation : Methanol significantly influences the reaction behavior of nitrogen during the supercritical water oxidation of ammonia, leading to increased conversion to N2O and NO (Oe et al., 2007).
Methanol Hydrocarbonation Process : This process refines raw material gas in combinative alkali ammonia synthesis plants, improving operating conditions and catalyst characteristics (Zhi, 2005).
Methanol and Ammonia as Clean Energy Sources : Methanol production provides a clean-burning fuel option, facilitates CO2 reduction, and offers hydrogen storage solutions. It has applications in DME, hydrogen, and DMFC fuel cells (Dalena et al., 2018).
Renewable Energy Production : Renewable methanol production using wind power-based hydrogen may be more economically viable than ammonia production, with additional environmental benefits (Matzen, Alhajji & Demirel, 2015).
Solid Oxide Membrane Reactors : These reactors demonstrate higher yields in hydrogenation reactions for ammonia and methanol synthesis compared to conventional catalytic reactors (Panagos, Voudouris & Stoukides, 1996).
Propriétés
IUPAC Name |
azane;methanol | |
---|---|---|
Details | Computed by LexiChem 2.6.6 (PubChem release 2019.06.18) | |
Source | PubChem | |
URL | https://pubchem.ncbi.nlm.nih.gov | |
Description | Data deposited in or computed by PubChem | |
InChI |
InChI=1S/CH4O.H3N/c1-2;/h2H,1H3;1H3 | |
Details | Computed by InChI 1.0.5 (PubChem release 2019.06.18) | |
Source | PubChem | |
URL | https://pubchem.ncbi.nlm.nih.gov | |
Description | Data deposited in or computed by PubChem | |
InChI Key |
CBHOOMGKXCMKIR-UHFFFAOYSA-N | |
Details | Computed by InChI 1.0.5 (PubChem release 2019.06.18) | |
Source | PubChem | |
URL | https://pubchem.ncbi.nlm.nih.gov | |
Description | Data deposited in or computed by PubChem | |
Canonical SMILES |
CO.N | |
Details | Computed by OEChem 2.1.5 (PubChem release 2019.06.18) | |
Source | PubChem | |
URL | https://pubchem.ncbi.nlm.nih.gov | |
Description | Data deposited in or computed by PubChem | |
Molecular Formula |
CH7NO | |
Details | Computed by PubChem 2.1 (PubChem release 2019.06.18) | |
Source | PubChem | |
URL | https://pubchem.ncbi.nlm.nih.gov | |
Description | Data deposited in or computed by PubChem | |
Molecular Weight |
49.073 g/mol | |
Details | Computed by PubChem 2.1 (PubChem release 2021.05.07) | |
Source | PubChem | |
URL | https://pubchem.ncbi.nlm.nih.gov | |
Description | Data deposited in or computed by PubChem | |
Product Name |
Ammonia methanol |
Synthesis routes and methods I
Procedure details
Synthesis routes and methods II
Procedure details
Synthesis routes and methods III
Procedure details
Synthesis routes and methods IV
Procedure details
Synthesis routes and methods V
Procedure details
Retrosynthesis Analysis
AI-Powered Synthesis Planning: Our tool employs the Template_relevance Pistachio, Template_relevance Bkms_metabolic, Template_relevance Pistachio_ringbreaker, Template_relevance Reaxys, Template_relevance Reaxys_biocatalysis model, leveraging a vast database of chemical reactions to predict feasible synthetic routes.
One-Step Synthesis Focus: Specifically designed for one-step synthesis, it provides concise and direct routes for your target compounds, streamlining the synthesis process.
Accurate Predictions: Utilizing the extensive PISTACHIO, BKMS_METABOLIC, PISTACHIO_RINGBREAKER, REAXYS, REAXYS_BIOCATALYSIS database, our tool offers high-accuracy predictions, reflecting the latest in chemical research and data.
Strategy Settings
Precursor scoring | Relevance Heuristic |
---|---|
Min. plausibility | 0.01 |
Model | Template_relevance |
Template Set | Pistachio/Bkms_metabolic/Pistachio_ringbreaker/Reaxys/Reaxys_biocatalysis |
Top-N result to add to graph | 6 |
Feasible Synthetic Routes
Avertissement et informations sur les produits de recherche in vitro
Veuillez noter que tous les articles et informations sur les produits présentés sur BenchChem sont destinés uniquement à des fins informatives. Les produits disponibles à l'achat sur BenchChem sont spécifiquement conçus pour des études in vitro, qui sont réalisées en dehors des organismes vivants. Les études in vitro, dérivées du terme latin "in verre", impliquent des expériences réalisées dans des environnements de laboratoire contrôlés à l'aide de cellules ou de tissus. Il est important de noter que ces produits ne sont pas classés comme médicaments et n'ont pas reçu l'approbation de la FDA pour la prévention, le traitement ou la guérison de toute condition médicale, affection ou maladie. Nous devons souligner que toute forme d'introduction corporelle de ces produits chez les humains ou les animaux est strictement interdite par la loi. Il est essentiel de respecter ces directives pour assurer la conformité aux normes légales et éthiques en matière de recherche et d'expérimentation.