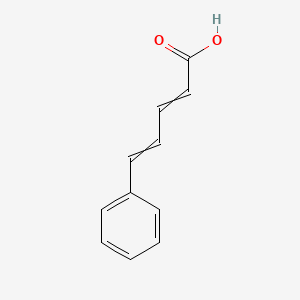
5-Phenylpenta-2,4-dienoic acid
Vue d'ensemble
Description
Méthodes De Préparation
Synthetic Routes and Reaction Conditions
5-Phenylpenta-2,4-dienoic acid can be synthesized through the esterification of hydroxyl or amine end groups with cinnamylidene acetic acid or cinnamylidene acetyl chloride . The reaction typically involves oligo(ethylene glycol) or oligo(propylene glycol) with varying molecular architectures and molecular weights. The degree of functionalization achieved in these reactions is generally higher than 90%.
Industrial Production Methods
While specific industrial production methods for cinnamylidene acetic acid are not extensively documented, the compound is available commercially and is often used in research settings. The production process likely involves similar esterification or amide formation reactions as described above, optimized for large-scale synthesis.
Analyse Des Réactions Chimiques
Types of Reactions
5-Phenylpenta-2,4-dienoic acid primarily undergoes photoinduced [2+2] cycloaddition reactions . This reaction is reversible and can be influenced by various factors such as light intensity and wavelength.
Common Reagents and Conditions
The photoinduced [2+2] cycloaddition reaction of cinnamylidene acetic acid typically requires ultraviolet light as the primary reagent. The reaction conditions include controlled light exposure and temperature to ensure the desired cycloaddition and minimize side reactions .
Major Products Formed
The major products formed from the photoinduced [2+2] cycloaddition of cinnamylidene acetic acid are cyclobutane derivatives. These products can further undergo photo-scission to revert to the original cinnamylidene acetic acid or form other by-products depending on the reaction conditions .
Applications De Recherche Scientifique
5-Phenylpenta-2,4-dienoic acid has several scientific research applications:
Chemistry: Used in the synthesis of photoresponsive polymers and materials.
Biology: Investigated for its potential antibacterial properties.
Medicine: Explored for its potential use in drug delivery systems due to its photoresponsive nature.
Industry: Utilized in the development of smart materials that can change properties in response to light.
Mécanisme D'action
The primary mechanism of action for cinnamylidene acetic acid involves its ability to undergo reversible [2+2] cycloaddition reactions under light exposure . This photoinduced reaction allows the compound to form and break cyclobutane rings, making it useful in applications requiring reversible changes in molecular structure. The molecular targets and pathways involved include the specific double bonds in the cinnamylidene acetic acid structure that participate in the cycloaddition reaction .
Comparaison Avec Des Composés Similaires
Similar Compounds
Cinnamylidene derivatives of rhodanine: These compounds have been investigated for their anthelmintic activity.
Cinnamylidene-thiazolidinedione derivatives: Explored for their potential as protein tyrosine phosphatase inhibitors.
2-thiohydantoin derivatives: Studied for their antifungal properties.
Uniqueness
5-Phenylpenta-2,4-dienoic acid is unique due to its high degree of photoresponsiveness and the ability to undergo reversible [2+2] cycloaddition reactions. This property makes it particularly valuable in the development of smart materials and photoresponsive polymers, setting it apart from other similar compounds that may not exhibit the same level of photoinduced reactivity .
Propriétés
IUPAC Name |
5-phenylpenta-2,4-dienoic acid | |
---|---|---|
Details | Computed by Lexichem TK 2.7.0 (PubChem release 2021.05.07) | |
Source | PubChem | |
URL | https://pubchem.ncbi.nlm.nih.gov | |
Description | Data deposited in or computed by PubChem | |
InChI |
InChI=1S/C11H10O2/c12-11(13)9-5-4-8-10-6-2-1-3-7-10/h1-9H,(H,12,13) | |
Details | Computed by InChI 1.0.6 (PubChem release 2021.05.07) | |
Source | PubChem | |
URL | https://pubchem.ncbi.nlm.nih.gov | |
Description | Data deposited in or computed by PubChem | |
InChI Key |
FEIQOMCWGDNMHM-UHFFFAOYSA-N | |
Details | Computed by InChI 1.0.6 (PubChem release 2021.05.07) | |
Source | PubChem | |
URL | https://pubchem.ncbi.nlm.nih.gov | |
Description | Data deposited in or computed by PubChem | |
Canonical SMILES |
C1=CC=C(C=C1)C=CC=CC(=O)O | |
Details | Computed by OEChem 2.3.0 (PubChem release 2021.05.07) | |
Source | PubChem | |
URL | https://pubchem.ncbi.nlm.nih.gov | |
Description | Data deposited in or computed by PubChem | |
Molecular Formula |
C11H10O2 | |
Details | Computed by PubChem 2.1 (PubChem release 2021.05.07) | |
Source | PubChem | |
URL | https://pubchem.ncbi.nlm.nih.gov | |
Description | Data deposited in or computed by PubChem | |
Molecular Weight |
174.20 g/mol | |
Details | Computed by PubChem 2.1 (PubChem release 2021.05.07) | |
Source | PubChem | |
URL | https://pubchem.ncbi.nlm.nih.gov | |
Description | Data deposited in or computed by PubChem | |
CAS No. |
1552-94-9 | |
Record name | 5-Phenyl-2,4-pentadienoic acid | |
Source | CAS Common Chemistry | |
URL | https://commonchemistry.cas.org/detail?cas_rn=1552-94-9 | |
Description | CAS Common Chemistry is an open community resource for accessing chemical information. Nearly 500,000 chemical substances from CAS REGISTRY cover areas of community interest, including common and frequently regulated chemicals, and those relevant to high school and undergraduate chemistry classes. This chemical information, curated by our expert scientists, is provided in alignment with our mission as a division of the American Chemical Society. | |
Explanation | The data from CAS Common Chemistry is provided under a CC-BY-NC 4.0 license, unless otherwise stated. | |
Synthesis routes and methods I
Procedure details
Synthesis routes and methods II
Procedure details
Synthesis routes and methods III
Procedure details
Synthesis routes and methods IV
Procedure details
Retrosynthesis Analysis
AI-Powered Synthesis Planning: Our tool employs the Template_relevance Pistachio, Template_relevance Bkms_metabolic, Template_relevance Pistachio_ringbreaker, Template_relevance Reaxys, Template_relevance Reaxys_biocatalysis model, leveraging a vast database of chemical reactions to predict feasible synthetic routes.
One-Step Synthesis Focus: Specifically designed for one-step synthesis, it provides concise and direct routes for your target compounds, streamlining the synthesis process.
Accurate Predictions: Utilizing the extensive PISTACHIO, BKMS_METABOLIC, PISTACHIO_RINGBREAKER, REAXYS, REAXYS_BIOCATALYSIS database, our tool offers high-accuracy predictions, reflecting the latest in chemical research and data.
Strategy Settings
Precursor scoring | Relevance Heuristic |
---|---|
Min. plausibility | 0.01 |
Model | Template_relevance |
Template Set | Pistachio/Bkms_metabolic/Pistachio_ringbreaker/Reaxys/Reaxys_biocatalysis |
Top-N result to add to graph | 6 |
Feasible Synthetic Routes
Avertissement et informations sur les produits de recherche in vitro
Veuillez noter que tous les articles et informations sur les produits présentés sur BenchChem sont destinés uniquement à des fins informatives. Les produits disponibles à l'achat sur BenchChem sont spécifiquement conçus pour des études in vitro, qui sont réalisées en dehors des organismes vivants. Les études in vitro, dérivées du terme latin "in verre", impliquent des expériences réalisées dans des environnements de laboratoire contrôlés à l'aide de cellules ou de tissus. Il est important de noter que ces produits ne sont pas classés comme médicaments et n'ont pas reçu l'approbation de la FDA pour la prévention, le traitement ou la guérison de toute condition médicale, affection ou maladie. Nous devons souligner que toute forme d'introduction corporelle de ces produits chez les humains ou les animaux est strictement interdite par la loi. Il est essentiel de respecter ces directives pour assurer la conformité aux normes légales et éthiques en matière de recherche et d'expérimentation.