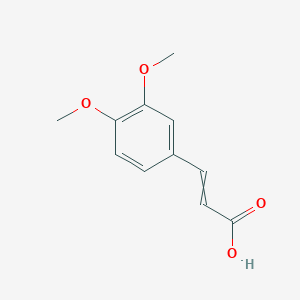
3,4-DIMETHOXYCINNAMIC ACID
Vue d'ensemble
Description
3,4-Dimethoxycinnamic acid (CAS: 2316-26-9; EINECS: 208-487-3) is a cinnamic acid derivative with methoxy (-OCH₃) groups at the 3 and 4 positions of the phenyl ring. Its molecular formula is C₁₁H₁₂O₄, with a molecular weight of 208.21 g/mol and a melting point of 181–183°C . The compound exists predominantly in the trans (E) isomeric form, though the cis (Z) isomer (CAS: 14737-88-3) has also been documented . It is widely utilized in organic synthesis for preparing amides and esters via coupling reactions and methylations .
Méthodes De Préparation
Knoevenagel-Doebner Condensation: Primary Synthetic Route
The Knoevenagel-Doebner reaction remains the most widely employed method for synthesizing 3,4-dimethoxycinnamic acid due to its high efficiency and straightforward protocol. This base-catalyzed condensation couples veratraldehyde (3,4-dimethoxybenzaldehyde) with malonic acid to form the α,β-unsaturated carboxylic acid scaffold.
Standard Reaction Conditions
A representative procedure involves heating veratraldehyde (500 mg, 1.0 equiv) and malonic acid (981 mg, 2.0 equiv) in dimethylformamide (DMF, 5 mL) with 1,4-diazabicyclo[2.2.2]octane (DABCO, 105 mg, 2 equiv) at 100–110°C for 60–90 minutes . The reaction progress is monitored via thin-layer chromatography (TLC), with typical Rf values of 0.35–0.45 in chloroform/methanol (9:1). Post-reaction workup includes extraction with ethyl acetate and recrystallization from chloroform/hexane, yielding colorless crystals (96% yield) .
Mechanistic Insights
The reaction proceeds through a nucleophilic attack by the enolate of malonic acid on the carbonyl carbon of veratraldehyde, followed by dehydration to form the conjugated double bond. DABCO acts as both a base and a mild catalyst, minimizing side reactions such as decarboxylation. The electron-donating methoxy groups on the aromatic ring enhance the electrophilicity of the aldehyde, facilitating condensation .
Alternative Synthesis via Catalytic Hydrogenation
A patent-published method (CN105601496B) describes an alternative route involving hydrogenation of preformed intermediates . While primarily designed for synthesizing 3,4-dimethoxybenzenepropanoic acid, this approach provides insights into modifications applicable to cinnamic acid derivatives.
Stepwise Hydrogenation Protocol
-
Initial Condensation : Veratraldehyde reacts with ethyl acetoacetate in ethyl acetate using sodium ethoxide as a base, forming the corresponding β-keto ester intermediate.
-
Selective Hydrogenation : The intermediate undergoes catalytic hydrogenation with 5% Pd/C under 3 atm H2 at 50°C, reducing the keto group while preserving the methoxy substituents.
-
Acid Hydrolysis : The ester is hydrolyzed with hydrochloric acid (10% v/v) at reflux to yield this compound .
Yield Optimization
This method achieves a 75% overall yield after recrystallization from ethanol/water (1:3). The use of sodium ethoxide instead of DABCO reduces side product formation but requires stringent moisture control .
Comparative Analysis of Synthetic Methods
The Knoevenagel-Doebner method offers higher yields and shorter reaction times, making it preferable for laboratory-scale synthesis. However, the hydrogenation route provides better scalability for industrial production due to milder conditions and easier catalyst recovery .
Purification and Characterization
Recrystallization Techniques
Recrystallization from chloroform/hexane (1:2) produces analytically pure crystals with a melting point of 131–133°C . Alternative solvent systems like ethanol/water (1:3) yield comparable purity but require higher volumes .
Spectroscopic Data
-
1H NMR (500 MHz, CDCl3) : δ 3.92 (s, 6H, OCH3), 6.66 (d, J = 15.3 Hz, 1H, CH=CH), 7.58 (d, J = 15.3 Hz, 1H, CH=CH), 6.74 (s, 2H, aromatic H) .
-
13C NMR (125 MHz, CDCl3) : δ 56.39 (OCH3), 116.82 (aromatic C), 144.21 (CH=CH), 168.44 (COOH) .
Industrial Applications and Modifications
Recent studies highlight this compound as a precursor for bioactive derivatives. For instance, thiomorpholine conjugates synthesized via DCC-mediated coupling exhibit potent antioxidant activity (IC50 = 8.2 μM against DPPH radicals) . Such derivatives retain the methoxy groups critical for membrane permeability, underscoring the compound’s versatility in drug design.
Analyse Des Réactions Chimiques
2.1. Phosphoric Acid-Catalyzed 1,4-Addition
DMCA undergoes 1,4-addition with electron-rich arenes (e.g., anisole, dimethoxybenzenes) in the presence of 85% H₃PO₄, forming 3,3-diarylpropionic acids .
Key Findings :
-
Reactivity : Methoxy or hydroxyl groups on the arene are essential for nucleophilic attack.
-
Yield : 85–95% for adducts with anisole or dimethoxybenzenes .
-
Mechanism :
Example Reaction :
Photochemical [2+2] Cycloaddition
DMCA exhibits solid-state photoreactivity, forming α-truxillic acid derivatives under UV light. The reaction efficiency depends on crystal packing .
Polymorph | Crystal System | Photoreactivity | Dimer Yield |
---|---|---|---|
Form I | Triclinic | Photosalient | ~40% |
Form II | Monoclinic | Non-photosalient | >80% |
Mechanism :
-
Topochemical Control : Alignment of DMCA molecules in Form II allows efficient [2+2] cycloaddition.
-
Photosalience : Form I’s mechanical stress during dimerization causes crystal fracturing .
Enzymatic Interesterification
DMCA’s ethyl ester reacts with phosphatidylcholine (PC) in lipase-catalyzed interesterification, yielding modified phospholipids .
Optimized Conditions :
-
Enzyme : Novozym 435 (30% w/w)
-
Solvent : Heptane
-
Molar Ratio (PC:Ester) : 1:10
Applications :
-
Enhanced bioavailability of DMCA in lipid-based drug delivery systems.
5.1. Esterification and Amidation
DMCA’s carboxylic acid group undergoes standard derivatization:
Reaction Type | Reagents | Product | Yield |
---|---|---|---|
Esterification | Cinnamyl alcohol, DCC/DMAP | Cinnamyl 3,4-dimethoxycinnamate | 82% |
Amidation | Thiomorpholine, DCC/DMAP | 3,4-Dimethoxycinnamoylthiomorpholide | 78% |
Conditions :
Biological Activity and Metabolic Stability
-
Antioxidant Activity : DMCA scavenges ROS via its methoxy groups, reducing oxidative stress in hepatocytes .
-
Metabolic Stability : Methoxy groups hinder Phase II metabolism (e.g., glucuronidation), enhancing bioavailability compared to hydroxylated analogs .
Comparison of Reactivity
Reaction Type | Conditions | Key Factor Influencing Reactivity |
---|---|---|
Knoevenagel-Doebner Synthesis | Basic, high temperature | Electron-withdrawing methoxy groups |
Photodimerization | UV light, solid state | Crystal packing (Polymorph-dependent) |
Enzymatic Interesterification | Lipase, non-polar solvent | Polarity of acyl acceptor |
Mechanistic Insights
Applications De Recherche Scientifique
Biological Properties
1. Antioxidant Activity
DMCA exhibits significant antioxidant properties, which can be attributed to its ability to scavenge free radicals. A study showed that DMCA demonstrated profound radical scavenging capability compared to other established antioxidants like indomethacin, suggesting its potential use in managing oxidative stress-related conditions .
2. Antidiabetic Effects
Research indicates that DMCA possesses strong antidiabetic activity. It has been shown to stimulate insulin secretion, improve pancreatic β-cell function, and inhibit gluconeogenesis in the liver. In vitro studies revealed that DMCA exhibited a 100-fold higher antidiabetic activity compared to the reference compound 1-deoxynojirimycin .
3. Hepatoprotective Properties
DMCA has demonstrated hepatoprotective effects in studies involving rat hepatocytes exposed to carbon tetrachloride (CCl₄). It was found to positively influence liver enzyme activity and improve oxidative stress balance, showing comparable efficacy to silybin at significantly lower concentrations .
Neuroprotective Applications
1. Inhibition of Prion Propagation
DMCA has been identified as a promising anti-prion agent with potential applications in neurodegenerative disease prevention. It binds effectively to prion proteins, reducing oligomer formation by 30-40% and enhancing cell viability in neuroblastoma models. This suggests that DMCA could serve as a dietary compound for the prophylaxis of neurodegenerative diseases such as prion diseases .
2. Memory Improvement
In vivo studies indicate that DMCA can significantly reduce memory deficits in rodent models of amnesia. The compound's efficacy surpassed that of velnakrine, a drug currently under investigation for Alzheimer’s disease treatment .
Anticancer Potential
DMCA has shown anticancer properties across various studies. Its ability to inhibit cancer cell proliferation and induce apoptosis has been documented, highlighting its potential as an adjunct therapy in cancer treatment .
Food Science Applications
In food science, DMCA is recognized for its health benefits when incorporated into food products. Its antioxidant properties can enhance the shelf life and nutritional profile of food items. The compound's bioavailability is a critical factor; methods such as lipophilization have been explored to improve its absorption and efficacy when consumed .
Case Studies
Case Study 1: Neuroprotective Effects
A study conducted on human neuroblastoma cells demonstrated that DMCA significantly reduced the formation of toxic prion protein oligomers while increasing cell viability by 30-40%. This study utilized various methodologies including isothermal titration calorimetry and dynamic light scattering to assess the binding affinity and protective effects of DMCA against neurotoxic agents .
Case Study 2: Antidiabetic Properties
In a controlled laboratory setting, DMCA was administered to diabetic rat models, resulting in marked improvements in glucose metabolism and insulin sensitivity compared to untreated controls. The findings support the potential use of DMCA as a therapeutic agent for managing diabetes .
Mécanisme D'action
The mechanism of action of 3,4-dimethoxycinnamic acid primarily involves its antioxidant activity. It can donate electrons to neutralize free radicals, thereby preventing oxidative damage to cells and tissues. The compound also inhibits the activity of enzymes like lipoxygenase and cyclooxygenase , which are involved in the inflammatory response . Additionally, it modulates lipid metabolism by influencing the expression of genes related to lipid synthesis and degradation .
Comparaison Avec Des Composés Similaires
Comparison with Structurally Similar Compounds
Structural and Physical Properties
The table below compares 3,4-dimethoxycinnamic acid with key analogs:
Key Observations:
- Substituent Effects : Methoxy groups in this compound reduce polarity compared to hydroxylated analogs like caffeic acid, enhancing lipid solubility and stability but reducing antioxidant activity .
- Isomerism : The trans isomer of this compound is more prevalent in commercial samples (99% purity) due to thermodynamic stability .
- Hydrogenation : Saturation of the α,β-unsaturated bond (e.g., 3,4-dimethoxyhydrocinnamic acid) lowers reactivity, making it suitable for controlled drug release .
Activité Biologique
3,4-Dimethoxycinnamic acid (DMCA) is a phenolic compound recognized for its diverse biological activities. This article delves into its antioxidant, anti-inflammatory, anticancer, and neuroprotective properties, supported by various studies and case analyses.
Chemical Structure and Properties
This compound is chemically defined as 3-(3,4-dimethoxyphenyl)propenoic acid. It features two methoxy groups at the 3 and 4 positions of the aromatic ring, which significantly contribute to its biological properties by enhancing binding affinity to various biological targets .
1. Antioxidant Activity
DMCA exhibits strong antioxidant properties, primarily due to the presence of methoxy groups that stabilize free radicals. In vitro studies have demonstrated its ability to scavenge reactive oxygen species (ROS), thereby reducing oxidative stress in cells .
2. Anti-inflammatory Effects
Research indicates that DMCA possesses notable anti-inflammatory effects. In a study comparing various compounds, DMCA showed significant inhibition of inflammatory markers when tested against standard drugs like ibuprofen . This activity is attributed to its ability to modulate pathways involved in inflammation.
3. Anticancer Properties
DMCA has been investigated for its anticancer potential across multiple studies. It has shown effectiveness in inhibiting cancer cell proliferation in various models:
In vivo studies have demonstrated that DMCA can penetrate biological membranes effectively, enhancing its therapeutic potential against colorectal adenocarcinoma cells .
4. Neuroprotective Effects
DMCA has shown promising results in neuroprotection. It significantly increased cell viability in neuroblastoma cells and reduced the formation of toxic prion protein oligomers by 30-40% compared to controls . This suggests potential applications in treating neurodegenerative diseases.
Case Studies
A notable case study involved the administration of DMCA in a rodent model exhibiting scopolamine-induced amnesia. Results indicated a reduction in memory deficits by approximately 60%, outperforming conventional treatments such as velnakrine used for Alzheimer's disease .
The mechanisms underlying the biological activities of DMCA are multifaceted:
- Antioxidant Mechanism: The methoxy groups enhance electron donation to free radicals, stabilizing them and preventing cellular damage.
- Anti-inflammatory Mechanism: DMCA modulates inflammatory pathways by inhibiting pro-inflammatory cytokines and enzymes such as COX-2.
- Anticancer Mechanism: DMCA induces apoptosis in cancer cells through the activation of caspases and modulation of cell cycle regulators.
- Neuroprotective Mechanism: Interaction with prion proteins reduces their aggregation and toxicity, thereby protecting neuronal health.
Q & A
Basic Research Questions
Q. What analytical techniques are recommended for characterizing 3,4-Dimethoxycinnamic acid, and how are they applied?
To confirm the identity and purity of this compound, researchers should employ a combination of spectroscopic and crystallographic methods:
- Mass Spectrometry (MS): Analyze trimethylsilyl derivatives to confirm molecular weight and fragmentation patterns .
- Nuclear Magnetic Resonance (NMR): Use H and C NMR to resolve methoxy and aromatic proton signals, critical for structural validation .
- X-ray Crystallography: Provides definitive confirmation of stereochemistry and intermolecular interactions, particularly for polymorphic studies .
- UV-Vis Spectroscopy: Useful for studying electronic transitions in solutions, aiding in purity assessments .
Q. What safety protocols are essential when handling this compound in laboratory settings?
The compound is toxic if ingested, causes skin/eye irritation, and may impair fertility. Key precautions include:
- Personal Protective Equipment (PPE): Gloves, lab coats, and safety goggles.
- Ventilation: Use fume hoods to avoid inhalation of aerosols.
- Waste Disposal: Follow hazardous waste guidelines for carboxylic acids due to their reactivity with bases .
Q. How is this compound utilized as a matrix in MALDI-MSI, and what advantages does it offer?
As a matrix for Matrix-Assisted Laser Desorption/Ionization Mass Spectrometry Imaging (MALDI-MSI), it enhances detection of low molecular weight (<500 Da) compounds by reducing background interference. Researchers should:
- Optimize Concentration: Use 10–20 mg/mL in organic solvents (e.g., acetonitrile) for homogeneous crystallization.
- Laser Energy Adjustment: Lower laser energy compared to traditional matrices (e.g., sinapic acid) to prevent analyte degradation .
Advanced Research Questions
Q. How do structural modifications (e.g., methoxy vs. hydroxyl groups) influence the biological activity of this compound?
Structure-Activity Relationship (SAR) studies highlight:
- Methoxy Substitution: The 3,4-dimethoxy configuration reduces osteogenic activity compared to hydroxylated analogs (e.g., p-hydroxycinnamic acid) but enhances antifungal properties in plant extracts .
- Stereoelectronic Effects: Methoxy groups increase lipophilicity, improving blood-brain barrier permeability for neuroactive applications .
Q. What microbial degradation pathways have been identified for this compound, and how can they inform bioremediation strategies?
- Fusarium spp. Biodegradation: Fusarium strains metabolize the compound via oxidative cleavage of the propenoic side chain, producing 3,4-dimethoxybenzoic acid as a intermediate. Researchers can track degradation using UV spectroscopy at 280 nm .
- Enzymatic Targets: Laccases and peroxidases are implicated in lignin-related aromatic compound breakdown, suggesting potential for engineered bioremediation .
Q. How can computational methods like molecular docking guide the development of this compound as a multi-target therapeutic agent?
- Virtual Screening: Dock the compound against Alzheimer’s disease targets (e.g., acetylcholinesterase, β-secretase) to prioritize experimental validation.
- Binding Affinity Analysis: Use software like AutoDock Vina to calculate ΔG values, focusing on hydrogen bonding with methoxy groups and π-π stacking with aromatic residues .
Q. How should researchers address contradictions in reported biological activities (e.g., antifungal vs. lack of osteogenic effects)?
- Contextual Factors: Evaluate differences in cell models (e.g., fungal vs. mammalian systems) and concentration ranges. For example, antifungal activity in plant extracts may require synergistic interactions with other phytochemicals .
- Dose-Response Studies: Conduct assays across a broad concentration spectrum (e.g., 1–100 μM) to identify therapeutic windows .
Q. What natural sources of this compound are available for phytochemical studies?
The compound is found in:
- Plant Extracts: Escallonia spp. and Cinnamomum cassia (Chinese cinnamon) peels. Ethanolic extraction followed by HPLC is recommended for isolation .
Q. Methodological Notes
- Data Reproducibility: Cross-validate spectroscopic results with multiple techniques (e.g., NMR + MS) to address variability in commercial samples .
- Toxicity Mitigation: Pre-screen derivatives for reduced toxicity using in silico tools like ProTox-II before in vitro testing .
Propriétés
IUPAC Name |
3-(3,4-dimethoxyphenyl)prop-2-enoic acid | |
---|---|---|
Details | Computed by LexiChem 2.6.6 (PubChem release 2019.06.18) | |
Source | PubChem | |
URL | https://pubchem.ncbi.nlm.nih.gov | |
Description | Data deposited in or computed by PubChem | |
InChI |
InChI=1S/C11H12O4/c1-14-9-5-3-8(4-6-11(12)13)7-10(9)15-2/h3-7H,1-2H3,(H,12,13) | |
Details | Computed by InChI 1.0.5 (PubChem release 2019.06.18) | |
Source | PubChem | |
URL | https://pubchem.ncbi.nlm.nih.gov | |
Description | Data deposited in or computed by PubChem | |
InChI Key |
HJBWJAPEBGSQPR-UHFFFAOYSA-N | |
Details | Computed by InChI 1.0.5 (PubChem release 2019.06.18) | |
Source | PubChem | |
URL | https://pubchem.ncbi.nlm.nih.gov | |
Description | Data deposited in or computed by PubChem | |
Canonical SMILES |
COC1=C(C=C(C=C1)C=CC(=O)O)OC | |
Details | Computed by OEChem 2.1.5 (PubChem release 2019.06.18) | |
Source | PubChem | |
URL | https://pubchem.ncbi.nlm.nih.gov | |
Description | Data deposited in or computed by PubChem | |
Molecular Formula |
C11H12O4 | |
Details | Computed by PubChem 2.1 (PubChem release 2019.06.18) | |
Source | PubChem | |
URL | https://pubchem.ncbi.nlm.nih.gov | |
Description | Data deposited in or computed by PubChem | |
DSSTOX Substance ID |
DTXSID10871869 | |
Record name | 3,4-Dimethoxycinnamic acid | |
Source | EPA DSSTox | |
URL | https://comptox.epa.gov/dashboard/DTXSID10871869 | |
Description | DSSTox provides a high quality public chemistry resource for supporting improved predictive toxicology. | |
Molecular Weight |
208.21 g/mol | |
Details | Computed by PubChem 2.1 (PubChem release 2021.05.07) | |
Source | PubChem | |
URL | https://pubchem.ncbi.nlm.nih.gov | |
Description | Data deposited in or computed by PubChem | |
CAS No. |
2316-26-9 | |
Record name | 3′,4′-Dimethoxycinnamic acid | |
Source | CAS Common Chemistry | |
URL | https://commonchemistry.cas.org/detail?cas_rn=2316-26-9 | |
Description | CAS Common Chemistry is an open community resource for accessing chemical information. Nearly 500,000 chemical substances from CAS REGISTRY cover areas of community interest, including common and frequently regulated chemicals, and those relevant to high school and undergraduate chemistry classes. This chemical information, curated by our expert scientists, is provided in alignment with our mission as a division of the American Chemical Society. | |
Explanation | The data from CAS Common Chemistry is provided under a CC-BY-NC 4.0 license, unless otherwise stated. | |
Record name | 3,4-Dimethoxycinnamic acid | |
Source | EPA DSSTox | |
URL | https://comptox.epa.gov/dashboard/DTXSID10871869 | |
Description | DSSTox provides a high quality public chemistry resource for supporting improved predictive toxicology. | |
Synthesis routes and methods I
Procedure details
Synthesis routes and methods II
Procedure details
Synthesis routes and methods III
Procedure details
Retrosynthesis Analysis
AI-Powered Synthesis Planning: Our tool employs the Template_relevance Pistachio, Template_relevance Bkms_metabolic, Template_relevance Pistachio_ringbreaker, Template_relevance Reaxys, Template_relevance Reaxys_biocatalysis model, leveraging a vast database of chemical reactions to predict feasible synthetic routes.
One-Step Synthesis Focus: Specifically designed for one-step synthesis, it provides concise and direct routes for your target compounds, streamlining the synthesis process.
Accurate Predictions: Utilizing the extensive PISTACHIO, BKMS_METABOLIC, PISTACHIO_RINGBREAKER, REAXYS, REAXYS_BIOCATALYSIS database, our tool offers high-accuracy predictions, reflecting the latest in chemical research and data.
Strategy Settings
Precursor scoring | Relevance Heuristic |
---|---|
Min. plausibility | 0.01 |
Model | Template_relevance |
Template Set | Pistachio/Bkms_metabolic/Pistachio_ringbreaker/Reaxys/Reaxys_biocatalysis |
Top-N result to add to graph | 6 |
Feasible Synthetic Routes
Avertissement et informations sur les produits de recherche in vitro
Veuillez noter que tous les articles et informations sur les produits présentés sur BenchChem sont destinés uniquement à des fins informatives. Les produits disponibles à l'achat sur BenchChem sont spécifiquement conçus pour des études in vitro, qui sont réalisées en dehors des organismes vivants. Les études in vitro, dérivées du terme latin "in verre", impliquent des expériences réalisées dans des environnements de laboratoire contrôlés à l'aide de cellules ou de tissus. Il est important de noter que ces produits ne sont pas classés comme médicaments et n'ont pas reçu l'approbation de la FDA pour la prévention, le traitement ou la guérison de toute condition médicale, affection ou maladie. Nous devons souligner que toute forme d'introduction corporelle de ces produits chez les humains ou les animaux est strictement interdite par la loi. Il est essentiel de respecter ces directives pour assurer la conformité aux normes légales et éthiques en matière de recherche et d'expérimentation.