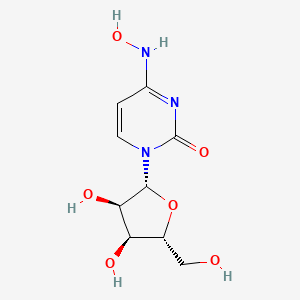
N(4)-Hydroxycytidine
Vue d'ensemble
Description
N(4)-Hydroxycytidine (NHC), also known as β-D-N4-hydroxycytidine, is a synthetic ribonucleoside analogue characterized by a hydroxyl group substituted at the N4 position of cytidine. This structural modification confers unique antiviral properties, enabling its role as a broad-spectrum inhibitor of RNA viruses, including SARS-CoV-2, alphaviruses (e.g., Chikungunya virus), and other coronaviruses .
Méthodes De Préparation
Hydroxylamination of Cytosine: Foundation of NHC Synthesis
The hydroxylamination of cytosine forms the cornerstone of NHC production, leveraging nucleophilic substitution at the N4 position. As detailed in patent CN113307833A, cytosine reacts with hydroxylamine derivatives (e.g., hydroxylamine hydrochloride or sulfate) under mildly acidic conditions (pH 5–7) at 55–65°C . Sodium acetate serves as both a buffer and catalyst, facilitating the displacement of the cytosine amino group by hydroxylamine.
Reaction Conditions and Yield Optimization
In Example 1 of the patent, 0.1 mol cytosine and 0.2 mol hydroxylamine hydrochloride in aqueous solution yielded 91.3% N4-hydroxycytosine after 3–5 hours . Ethanol co-solvents (water:ethanol = 4:1) further enhanced yields to 93.7% by improving hydroxylamine solubility . The reaction’s regioselectivity is attributed to the electron-withdrawing effect of the cytosine ring, which polarizes the N4 position for nucleophilic attack.
Silanization and Condensation with Tetraacetyl Ribose
Following hydroxylamination, the N4-hydroxycytosine intermediate undergoes silanization to protect reactive hydroxyl groups before ribose coupling. Triethylsilyl (TES) or triisopropylsilyl (TIPS) chlorides are commonly employed, forming silyl ether derivatives that prevent undesired side reactions during condensation .
Lewis Acid-Catalyzed Glycosylation
Condensation with 1,2,3,5-tetra-O-acetyl-β-D-ribofuranose (tetraacetyl ribose) occurs in the presence of Lewis acids such as BF3·Et2O or SnCl4 . These catalysts activate the ribose anomeric carbon, enabling nucleophilic attack by the N4-hydroxycytosine’s N1 atom. The reaction proceeds at 60–80°C in anhydrous acetonitrile, yielding triacetyl-N4-hydroxycytidine with >85% efficiency .
Acidic and Alkaline Deprotection Strategies
Final deprotection steps remove acetyl and silyl groups to yield free NHC. Two primary methodologies dominate:
Alkaline Hydrolysis
The patent method employs 0.1 M NaOH at 25°C for 12 hours, cleaving acetyl groups without degrading the ribose ring . This approach minimizes epimerization risks, preserving the β-D-configuration critical for antiviral activity .
Catalytic Hydrogenolysis
Alternative methods utilize Pd/C-catalyzed hydrogenolysis with cyclohexadiene as a hydrogen donor . For example, deprotection of benzyloxy-protected intermediates (e.g., compound 6 in PMC9504122) achieves quantitative yields under mild conditions (25°C, 6 hours) .
Phosphoramidite Synthesis for Oligonucleotide Incorporation
NHC’s integration into RNA oligonucleotides, as studied in SARS-CoV-2 mutagenesis research, requires specialized phosphoramidite derivatives .
Solid-Phase Synthesis
Protected NHC phosphoramidites (e.g., compound 3 ) are synthesized via sequential:
-
Benzoylation : N4-hydroxycytidine reacts with benzoic anhydride in CH2Cl2/DMAP, achieving 80% benzoyl protection .
-
Phosphitylation : Treatment with 2-cyanoethyl-N,N,N′,N′-tetraisopropylphosphorodiamidite and 4,5-dicyanoimidazole yields the active phosphoramidite (40% yield) .
This method enables automated RNA synthesis, critical for structural studies of viral RNA-dependent RNA polymerase (RdRp) interactions .
5′-O-Esterification via Steglich Reaction
Recent advances focus on modifying NHC’s 5′-hydroxyl to enhance pharmacokinetics. MDPI-reported Steglich esterification employs DCC/DMAP to couple carboxylic acids with NHC .
Representative Derivatives
Derivative | Carboxylic Acid | Yield (%) |
---|---|---|
5′-O-(4-Methylphenyl)acetyl | 4-Methylphenylacetic acid | 42 |
5′-O-(4-Fluorophenyl)acetyl | 4-Fluorophenylacetic acid | 42 |
5′-O-(2-Chlorophenyl)acetyl | 2-Chlorophenylacetic acid | 43 |
Reactions proceed in CH2Cl2 at 25°C, with purification via silica gel chromatography (CHCl3:MeOH) .
Comparative Analysis of Methodologies
Analyse Des Réactions Chimiques
La N4-Hydroxycytidine subit plusieurs types de réactions chimiques :
Mutagénèse : Le composé induit des mutations dans l'ARN viral, conduisant à une accumulation d'erreurs qui inhibent la réplication virale.
Les réactifs couramment utilisés dans ces réactions comprennent l'hydroxylamine, les ions cuivre et divers solvants . Les principaux produits formés sont généralement des nucléotides modifiés qui perturbent la réplication virale .
4. Applications de la Recherche Scientifique
La N4-Hydroxycytidine a une large gamme d'applications dans la recherche scientifique :
Biologie : Le composé est utilisé dans la recherche sur la réplication virale et la mutagénèse.
Industrie : Ses propriétés antivirales en font un composé précieux pour le développement de nouvelles thérapies antivirales.
5. Mécanisme d'Action
La N4-Hydroxycytidine exerce ses effets en étant phosphorylée dans les tissus en sa forme active 5'-triphosphate. Le composé cible les ARN polymérases dépendantes de l'ARN, conduisant à la perturbation de la synthèse de l'ARN viral .
Applications De Recherche Scientifique
Antiviral Mechanism of Action
NHC acts primarily by:
- Incorporation into Viral RNA : Once inside an infected cell, NHC is phosphorylated to its active form (NHC-TP) and incorporated into the viral RNA by RNA-dependent RNA polymerase (RdRp). This incorporation leads to erroneous base pairing during replication .
- Induction of Mutagenesis : The incorporation of NHC results in a high mutation rate within the viral genome, which can overwhelm the virus's ability to maintain a stable genome and propagate effectively .
- Broad-Spectrum Activity : NHC exhibits antiviral activity against various RNA viruses, including coronaviruses (e.g., SARS-CoV-2), influenza viruses, and bunyaviruses .
Treatment of COVID-19
NHC has been extensively studied for its role in treating COVID-19. Clinical trials have demonstrated that molnupiravir, as a prodrug of NHC, can reduce viral load and improve clinical outcomes in patients with COVID-19. Notably:
- Efficacy in Reducing Hospitalization : Studies indicate that patients treated with molnupiravir had lower rates of hospitalization compared to those receiving placebo .
- Mechanism Against Variants : Research shows that NHC can effectively inhibit SARS-CoV-2 variants by inducing mutations that hinder viral replication .
Other Viral Infections
Beyond COVID-19, NHC has shown potential against several other viral pathogens:
- Influenza : NHC demonstrated significant antiviral activity against both influenza A and B viruses, providing a promising avenue for treatment options where traditional antivirals may fail .
- Bunyaviruses : In animal models, NHC has been effective against La Crosse virus and other bunyaviruses, reducing neurological disease progression significantly .
Case Study 1: Efficacy Against La Crosse Virus
In a controlled study involving mice infected with La Crosse virus:
- Treatment Regimen : Mice were administered molnupiravir starting on the day of infection.
- Outcomes : The treatment led to a 31% reduction in neurological disease development and significantly lower viral titers in the central nervous system compared to untreated controls .
Case Study 2: Resistance Development
A study examining the emergence of resistance in SARS-CoV-2 variants highlighted:
- Mutation Patterns : Deep sequencing revealed that exposure to NHC accelerated the emergence of mutations associated with immune escape.
- Clinical Implications : These findings underscore the need for continuous monitoring of viral mutations in response to antiviral therapies like NHC .
Pharmacokinetics
The pharmacokinetic profile of NHC indicates variable absorption and distribution:
- Bioavailability : While NHC shows good oral bioavailability in mice, studies suggest limited bioavailability in non-human primates, which may impact clinical efficacy .
- Tissue Distribution : Concentrations of NHC have been measured in saliva and nasal secretions during clinical trials, indicating potential effectiveness at sites of viral entry .
Mécanisme D'action
N4-Hydroxycytidine exerts its effects by being phosphorylated in tissues to its active 5’-triphosphate form. The compound targets RNA-dependent RNA polymerases, leading to the disruption of viral RNA synthesis .
Comparaison Avec Des Composés Similaires
Mechanism of Action
NHC exerts its antiviral activity through lethal mutagenesis. Upon intracellular phosphorylation to its triphosphate form (NHC-TP), it incorporates into viral RNA during replication, causing mismatches and accumulation of errors that impair viral viability. Notably, NHC remains effective against coronaviruses with intact proofreading machinery (e.g., SARS-CoV-2), demonstrating a high genetic barrier to resistance .
Antiviral Spectrum and Efficacy
- SARS-CoV-2 : In vitro IC50 of 0.3 µM with a therapeutic index >100 (CC50 >10 µM) .
- Alphaviruses: Potent inhibition of Chikungunya virus (CHIKV) and other alphaviruses by inducing high mutation rates in viral genomes .
Structural Analogs: N4-Modified Cytidine Derivatives
N4-Acetylcytidine
- Structural Modification : N4-acetyl group.
- Applications: Limited data on antiviral activity; primarily studied in RNA modification contexts .
N4-Allylcytidine (a4C)
- Structural Modification : N4-allyl group.
- Applications : Used for RNA labeling and chemical sequencing. Iodination induces cyclization to form 3,N4-cyclic cytidine derivatives, enabling RNA structural studies .
- Key Difference : The allyl group facilitates chemical reactions for sequencing rather than antiviral activity, underscoring divergent functional outcomes of N4 modifications.
Functional Analogs: Antiviral Nucleoside Analogues
Molnupiravir (Prodrug of NHC)
- Role : Orally administered prodrug of NHC, enhancing bioavailability and therapeutic utility against SARS-CoV-2 .
- Differentiation : While NHC itself requires metabolic activation, Molnupiravir’s design optimizes pharmacokinetics for clinical use.
Ribavirin
- However, NHC demonstrates superior efficacy against proofreading-intact coronaviruses and a higher genetic barrier to resistance .
Data Tables
Table 1: Structural and Functional Comparison of N4-Modified Cytidine Analogues
Table 2: Antiviral Activity Profile of this compound
Activité Biologique
N(4)-Hydroxycytidine (NHC) is a nucleoside analogue that has garnered significant attention due to its broad-spectrum antiviral activity against various RNA viruses, including coronaviruses and influenza viruses. This article explores the biological activity of NHC, focusing on its mechanisms of action, pharmacological properties, and relevant case studies.
Overview of this compound
NHC is a small molecule that functions as a ribonucleoside analogue. It is particularly noted for its role in the treatment of viral infections, including those caused by SARS-CoV-2, the virus responsible for COVID-19. The compound is phosphorylated in tissues to form its active triphosphate form, which is then incorporated into viral RNA during replication, leading to an accumulation of mutations that can inhibit viral propagation—a phenomenon known as "viral error catastrophe" .
The antiviral activity of NHC is primarily attributed to its ability to induce lethal mutagenesis in viral populations. By mimicking natural nucleotides, NHC integrates into the viral RNA and causes errors during replication. This results in a high mutation rate that ultimately leads to viral extinction .
- Target Viruses : NHC has shown efficacy against a range of viruses:
- Coronaviruses : Effective against SARS-CoV-2, MERS-CoV, and murine hepatitis virus (MHV).
- Influenza Viruses : Inhibits various strains including H1N1 and H3N2.
- Other RNA Viruses : Demonstrated activity against Venezuelan equine encephalitis virus (VEEV), Ebola virus, and respiratory syncytial virus (RSV) .
Pharmacokinetics
Absorption and Bioavailability :
- NHC exhibits oral bioavailability in mice but is poorly bioavailable in non-human primates . This discrepancy highlights the need for further research into its pharmacokinetic properties across different species.
Distribution and Metabolism :
- After administration, NHC distributes into tissues where it is phosphorylated to its active form. The metabolic pathways include hydrolytic deamination leading to uridine formation .
Antiviral Efficacy Against SARS-CoV-2
Recent studies have demonstrated that NHC effectively inhibits SARS-CoV-2 replication. For instance:
- Study 1 : In vitro testing revealed an EC50 value (the concentration required for 50% inhibition of viral replication) of approximately 16.5 µM for NHC against SARS-CoV-2 .
- Study 2 : A novel analog of NHC, β-D-N4-O-isobutyrylcytidine (compound 8a), exhibited enhanced antiviral activity with an EC50 of 3.50 µM against SARS-CoV-2, outperforming both NHC and remdesivir .
Broader Spectrum Activity
NHC has been shown to inhibit a variety of RNA viruses beyond coronaviruses:
- Influenza Viruses : In vitro studies indicated that β-D-N4-O-isobutyrylcytidine also had potent activity against influenza A (H1N1 and H3N2) and B viruses with EC50 values ranging from 3.40 µM to 7.30 µM .
- Dengue Virus : The same compound showed promising results against Dengue virus serotype 2 (DENV-2) with an EC50 value of 3.95 µM .
Comparative Summary of Antiviral Activities
Compound | Virus Type | EC50 (µM) | Cytotoxicity CC50 (µM) |
---|---|---|---|
This compound | SARS-CoV-2 | >16.5 | >100 |
β-D-N4-O-isobutyrylcytidine | SARS-CoV-2 | 3.50 | >100 |
β-D-N4-O-isobutyrylcytidine | Influenza A H1N1 | 5.80 | >100 |
β-D-N4-O-isobutyrylcytidine | Influenza B | 3.40 | >100 |
β-D-N4-O-isobutyrylcytidine | DENV-2 | 3.95 | >100 |
Q & A
Basic Research Questions
Q. What is the primary antiviral mechanism of N(4)-Hydroxycytidine (NHC), and how does it differ from other nucleoside analogs?
NHC exerts its antiviral activity by acting as a mutagenic ribonucleoside analog. It incorporates into viral RNA during replication, inducing lethal mutagenesis through tautomerism and rotational isomerism. This allows NHC to mimic both cytidine and uridine, leading to mismatched base pairing and increased error rates in viral genomes. Unlike ribavirin, which primarily inhibits viral polymerases, NHC’s mutagenic mechanism reduces viral infectivity and replication efficiency across multiple RNA virus families, including coronaviruses and alphaviruses .
Q. How should researchers select appropriate in vitro models to evaluate NHC’s efficacy against RNA viruses?
Prioritize models that replicate human pathogenesis, such as:
- Human respiratory epithelial cells (e.g., Calu-3) for coronaviruses .
- Vero E6 cells for broad-spectrum RNA viruses (e.g., SARS-CoV-2, VEEV) .
- Primary cell cultures to assess tissue-specific toxicity (e.g., hepatocytes for metabolic studies). Validate models using viral load quantification (qRT-PCR) and plaque reduction assays to determine IC50 and CC50 values .
Q. What safety protocols are critical when handling NHC in laboratory settings?
Adhere to GHS hazard guidelines (acute toxicity, skin/eye irritation):
- Engineering controls : Use fume hoods and ensure adequate ventilation to avoid aerosol exposure .
- Personal protective equipment (PPE) : Wear nitrile gloves, lab coats, and safety goggles; respirators are recommended for powder handling .
- Emergency measures : Maintain accessible eyewash stations and safety showers. Decontaminate spills with ethanol/water mixtures and dispose of waste per local regulations .
Advanced Research Questions
Q. How can researchers experimentally quantify NHC-induced mutation rates in viral genomes?
Use deep sequencing (e.g., Illumina MiSeq) to analyze mutation frequencies in viral RNA extracted from treated vs. untreated cultures. Key steps:
- Amplify viral RNA regions of interest (e.g., polymerase genes).
- Compare transition/transversion ratios and identify "error catastrophe" thresholds (e.g., >3-fold increase in mutations at 1 µM NHC) .
- Validate findings with plaque assays to correlate mutation rates with loss of infectivity .
Q. What experimental designs are optimal for studying low-level resistance to NHC in alphaviruses?
Conduct serial passaging of viruses (e.g., VEEV) under sublethal NHC concentrations:
- Propagate virus in cell culture with incremental NHC doses (e.g., 0.1–5 µM) over 20–30 passages .
- Sequence viral genomes (e.g., nsP4 polymerase) to identify resistance-conferring mutations.
- Key finding : Resistance requires cooperative mutations (e.g., >2 mutations in nsP4), making it evolutionarily costly .
Q. How can NHC be integrated into combination therapies to mitigate antiviral resistance?
Pair NHC with non-mutagenic inhibitors (e.g., protease or entry inhibitors) to exploit synergistic effects:
- Example : Co-administer NHC with remdesivir in SARS-CoV-2 models, using Bliss independence or Loewe additivity models to quantify synergy .
- Monitor for cross-resistance using reverse genetics to engineer recombinant viruses with known resistance mutations .
Q. Methodological Guidance
Q. What statistical approaches are recommended for analyzing NHC’s dose-response relationships?
- Fit data to 4-parameter logistic curves (e.g., GraphPad Prism) to calculate EC50/EC90.
- Use ANOVA with post-hoc tests (e.g., Tukey’s) to compare viral loads across treatment groups.
- Report 95% confidence intervals and p-values for reproducibility assessments .
Q. How should researchers address contradictory data on NHC’s therapeutic index (TI) across studies?
- Re-evaluate experimental conditions : Differences in cell viability assays (e.g., MTT vs. ATP-based) may affect CC50 calculations .
- Standardize protocols: Use identical viral strains (e.g., SARS-CoV-2 USA-WA1/2020) and multiplicity of infection (MOI) values.
- Cross-validate findings with in vivo models (e.g., ferrets for respiratory viruses) .
Propriétés
IUPAC Name |
1-[(2R,3R,4S,5R)-3,4-dihydroxy-5-(hydroxymethyl)oxolan-2-yl]-4-(hydroxyamino)pyrimidin-2-one | |
---|---|---|
Source | PubChem | |
URL | https://pubchem.ncbi.nlm.nih.gov | |
Description | Data deposited in or computed by PubChem | |
InChI |
InChI=1S/C9H13N3O6/c13-3-4-6(14)7(15)8(18-4)12-2-1-5(11-17)10-9(12)16/h1-2,4,6-8,13-15,17H,3H2,(H,10,11,16)/t4-,6-,7-,8-/m1/s1 | |
Source | PubChem | |
URL | https://pubchem.ncbi.nlm.nih.gov | |
Description | Data deposited in or computed by PubChem | |
InChI Key |
XCUAIINAJCDIPM-XVFCMESISA-N | |
Source | PubChem | |
URL | https://pubchem.ncbi.nlm.nih.gov | |
Description | Data deposited in or computed by PubChem | |
Canonical SMILES |
C1=CN(C(=O)N=C1NO)C2C(C(C(O2)CO)O)O | |
Source | PubChem | |
URL | https://pubchem.ncbi.nlm.nih.gov | |
Description | Data deposited in or computed by PubChem | |
Isomeric SMILES |
C1=CN(C(=O)N=C1NO)[C@H]2[C@@H]([C@@H]([C@H](O2)CO)O)O | |
Source | PubChem | |
URL | https://pubchem.ncbi.nlm.nih.gov | |
Description | Data deposited in or computed by PubChem | |
Molecular Formula |
C9H13N3O6 | |
Source | PubChem | |
URL | https://pubchem.ncbi.nlm.nih.gov | |
Description | Data deposited in or computed by PubChem | |
DSSTOX Substance ID |
DTXSID20186274 | |
Record name | N(4)-Hydroxycytidine | |
Source | EPA DSSTox | |
URL | https://comptox.epa.gov/dashboard/DTXSID20186274 | |
Description | DSSTox provides a high quality public chemistry resource for supporting improved predictive toxicology. | |
Molecular Weight |
259.22 g/mol | |
Source | PubChem | |
URL | https://pubchem.ncbi.nlm.nih.gov | |
Description | Data deposited in or computed by PubChem | |
CAS No. |
3258-02-4 | |
Record name | N4-Hydroxycytidine | |
Source | CAS Common Chemistry | |
URL | https://commonchemistry.cas.org/detail?cas_rn=3258-02-4 | |
Description | CAS Common Chemistry is an open community resource for accessing chemical information. Nearly 500,000 chemical substances from CAS REGISTRY cover areas of community interest, including common and frequently regulated chemicals, and those relevant to high school and undergraduate chemistry classes. This chemical information, curated by our expert scientists, is provided in alignment with our mission as a division of the American Chemical Society. | |
Explanation | The data from CAS Common Chemistry is provided under a CC-BY-NC 4.0 license, unless otherwise stated. | |
Record name | N(4)-Hydroxycytidine | |
Source | ChemIDplus | |
URL | https://pubchem.ncbi.nlm.nih.gov/substance/?source=chemidplus&sourceid=0003258024 | |
Description | ChemIDplus is a free, web search system that provides access to the structure and nomenclature authority files used for the identification of chemical substances cited in National Library of Medicine (NLM) databases, including the TOXNET system. | |
Record name | N4-Hydroxycytidine | |
Source | DrugBank | |
URL | https://www.drugbank.ca/drugs/DB15660 | |
Description | The DrugBank database is a unique bioinformatics and cheminformatics resource that combines detailed drug (i.e. chemical, pharmacological and pharmaceutical) data with comprehensive drug target (i.e. sequence, structure, and pathway) information. | |
Explanation | Creative Common's Attribution-NonCommercial 4.0 International License (http://creativecommons.org/licenses/by-nc/4.0/legalcode) | |
Record name | N(4)-Hydroxycytidine | |
Source | EPA DSSTox | |
URL | https://comptox.epa.gov/dashboard/DTXSID20186274 | |
Description | DSSTox provides a high quality public chemistry resource for supporting improved predictive toxicology. | |
Record name | 1-[(2R,3R,4S,5R)-3,4-dihydroxy-5-(hydroxymethyl)oxolan-2-yl]-4-(hydroxyimino)-1,2,3,4-tetrahydropyrimidin-2-one | |
Source | European Chemicals Agency (ECHA) | |
URL | https://echa.europa.eu/information-on-chemicals | |
Description | The European Chemicals Agency (ECHA) is an agency of the European Union which is the driving force among regulatory authorities in implementing the EU's groundbreaking chemicals legislation for the benefit of human health and the environment as well as for innovation and competitiveness. | |
Explanation | Use of the information, documents and data from the ECHA website is subject to the terms and conditions of this Legal Notice, and subject to other binding limitations provided for under applicable law, the information, documents and data made available on the ECHA website may be reproduced, distributed and/or used, totally or in part, for non-commercial purposes provided that ECHA is acknowledged as the source: "Source: European Chemicals Agency, http://echa.europa.eu/". Such acknowledgement must be included in each copy of the material. ECHA permits and encourages organisations and individuals to create links to the ECHA website under the following cumulative conditions: Links can only be made to webpages that provide a link to the Legal Notice page. | |
Record name | N4-HYDROXYCYTIDINE | |
Source | FDA Global Substance Registration System (GSRS) | |
URL | https://gsrs.ncats.nih.gov/ginas/app/beta/substances/C3D11PV2O4 | |
Description | The FDA Global Substance Registration System (GSRS) enables the efficient and accurate exchange of information on what substances are in regulated products. Instead of relying on names, which vary across regulatory domains, countries, and regions, the GSRS knowledge base makes it possible for substances to be defined by standardized, scientific descriptions. | |
Explanation | Unless otherwise noted, the contents of the FDA website (www.fda.gov), both text and graphics, are not copyrighted. They are in the public domain and may be republished, reprinted and otherwise used freely by anyone without the need to obtain permission from FDA. Credit to the U.S. Food and Drug Administration as the source is appreciated but not required. | |
Avertissement et informations sur les produits de recherche in vitro
Veuillez noter que tous les articles et informations sur les produits présentés sur BenchChem sont destinés uniquement à des fins informatives. Les produits disponibles à l'achat sur BenchChem sont spécifiquement conçus pour des études in vitro, qui sont réalisées en dehors des organismes vivants. Les études in vitro, dérivées du terme latin "in verre", impliquent des expériences réalisées dans des environnements de laboratoire contrôlés à l'aide de cellules ou de tissus. Il est important de noter que ces produits ne sont pas classés comme médicaments et n'ont pas reçu l'approbation de la FDA pour la prévention, le traitement ou la guérison de toute condition médicale, affection ou maladie. Nous devons souligner que toute forme d'introduction corporelle de ces produits chez les humains ou les animaux est strictement interdite par la loi. Il est essentiel de respecter ces directives pour assurer la conformité aux normes légales et éthiques en matière de recherche et d'expérimentation.