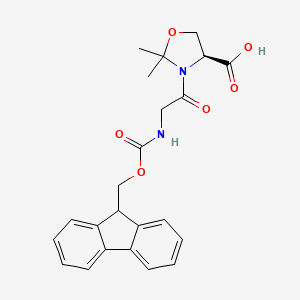
Fmoc-Gly-Ser(Psi(Me,Me)Pro)-OH
Vue d'ensemble
Description
Fmoc-Gly-Ser(Ψ(Me,Me)Pro)-OH is a pseudoproline dipeptide building block widely employed in solid-phase peptide synthesis (SPPS) under Fmoc chemistry. This compound incorporates a serine residue modified with a pseudoproline motif (Ψ(Me,Me)Pro), which disrupts β-sheet and α-helix formation during peptide elongation, thereby mitigating aggregation and improving synthesis efficiency . Its molecular formula is C₂₃H₂₄N₂O₆ (MW: 424.45 g/mol), and it is typically supplied as a white to yellow powder with solubility in polar aprotic solvents like DMF and DMSO .
Pseudoprolines are designed as oxazolidine derivatives, where the Ser/Thr sidechain hydroxyl group forms a cyclic structure with a dipeptide’s adjacent amino acid. In Fmoc-Gly-Ser(Ψ(Me,Me)Pro)-OH, the Gly-Ser sequence is conformationally constrained, reducing steric clashes and enabling smoother chain assembly in challenging sequences, such as those in insulin lispro and human growth hormone (hGH) derivatives . Upon cleavage with trifluoroacetic acid (TFA), the oxazolidine ring opens, regenerating the native Ser residue .
Méthodes De Préparation
Synthetic Routes and Reaction Conditions
The synthesis of Fmoc-Gly-Ser(Psi(Me,Me)Pro)-OH typically involves solid-phase peptide synthesis (SPPS) using Fmoc chemistry. The process begins with the anchoring of the first amino acid (Glycine) to a solid support resin. The Fmoc group is then removed using a solution of piperidine in dimethylformamide (DMF), exposing the amino group for subsequent coupling reactions.
The serine residue is then coupled to the glycine using a coupling reagent such as N,N’-diisopropylcarbodiimide (DIC) and 1-hydroxybenzotriazole (HOBt). The pseudo-proline residue is introduced in a similar manner, with the Fmoc group being removed and the residue being coupled to the growing peptide chain.
Industrial Production Methods
In an industrial setting, the synthesis of This compound can be automated using peptide synthesizers. These machines can perform the repetitive cycles of deprotection, washing, and coupling required for SPPS. The use of automated systems allows for the efficient and high-throughput production of peptides with high purity and yield.
Analyse Des Réactions Chimiques
Types of Reactions
Fmoc-Gly-Ser(Psi(Me,Me)Pro)-OH: can undergo various chemical reactions, including:
Oxidation: The serine residue can be oxidized to form a serine oxide derivative.
Reduction: The pseudo-proline residue can be reduced to form a proline derivative.
Substitution: The Fmoc group can be substituted with other protective groups or functional groups.
Common Reagents and Conditions
Oxidation: Oxidizing agents such as hydrogen peroxide (H2O2) or sodium periodate (NaIO4) can be used.
Reduction: Reducing agents such as sodium borohydride (NaBH4) or lithium aluminum hydride (LiAlH4) can be used.
Substitution: Substitution reactions can be carried out using various nucleophiles under basic conditions.
Major Products Formed
Oxidation: Serine oxide derivatives.
Reduction: Proline derivatives.
Substitution: Various substituted peptides with different functional groups.
Applications De Recherche Scientifique
Peptide Synthesis
Role as a Building Block:
Fmoc-Gly-Ser(Psi(Me,Me)Pro)-OH serves as a crucial building block in the synthesis of peptides. It is particularly utilized in solid-phase peptide synthesis (SPPS), where it facilitates the creation of specific peptide sequences. The incorporation of this compound allows for the production of complex peptides with high purity and yields, essential for various applications in drug development and biotechnology .
Overcoming Aggregation:
The presence of the pseudo-proline residue (Psi(Me,Me)Pro) helps to disrupt β-sheet structures that often lead to intermolecular aggregation during peptide chain elongation. This characteristic significantly enhances solvation and coupling kinetics, enabling researchers to access larger peptides through convergent strategies and chemoselective ligation techniques .
Drug Design
Peptide-Based Therapeutics:
The unique structure of this compound aids in the design of peptide-based drugs that target specific biological pathways. This capability enhances therapeutic efficacy while reducing potential side effects associated with traditional small molecule drugs. The compound's stability also contributes to the development of long-lasting therapeutic agents .
Targeted Drug Delivery:
In drug formulation, this compound is employed in bioconjugation processes that facilitate the attachment of peptides to other biomolecules, such as antibodies. This method is crucial for creating targeted delivery systems that improve the precision of therapeutic interventions .
Bioconjugation
Bioconjugation using this compound allows for the strategic linking of peptides with various biomolecules. This technique is vital for developing novel targeted therapies and enhancing the delivery mechanisms of therapeutic agents. The ability to modify peptides through bioconjugation broadens their application in clinical settings .
Research in Protein Engineering
Modification of Proteins:
this compound plays a significant role in protein engineering studies. Researchers utilize this compound to modify proteins for improved stability and functionality. Such modifications are essential for various industrial applications where protein performance is critical .
Exploration of Structure-Function Relationships:
The incorporation of this compound into protein structures allows scientists to explore intricate structure-function relationships, leading to the development of novel protein therapeutics that can be tailored for specific applications .
Analytical Chemistry
In analytical chemistry, this compound is utilized to study peptide interactions and molecular behavior. Its application aids in the development of new analytical techniques that provide insights into peptide dynamics and interactions at the molecular level .
Mécanisme D'action
The mechanism of action of Fmoc-Gly-Ser(Psi(Me,Me)Pro)-OH involves its incorporation into peptide chains during SPPS. The Fmoc group protects the amino group of the glycine residue, preventing unwanted side reactions during peptide synthesis. The pseudo-proline residue enhances the stability and solubility of the peptide, making it easier to handle and purify.
Comparaison Avec Des Composés Similaires
Pseudoproline dipeptides vary based on the amino acid residues and protecting groups. Below is a detailed comparison of Fmoc-Gly-Ser(Ψ(Me,Me)Pro)-OH with analogous compounds:
Structural and Functional Differences
Key Observations :
- Steric Effects : Leu-containing pseudoprolines (e.g., Fmoc-Leu-Ser(Ψ(Me,Me)Pro)-OH) introduce bulkier sidechains, which may hinder coupling in tightly packed sequences but are advantageous in disrupting hydrophobic aggregates .
- Solubility : Fmoc-Gly-Ser(Ψ(Me,Me)Pro)-OH’s smaller glycine residue enhances solubility in SPPS solvents compared to Ala or Leu variants, which may require extended coupling times .
Case Studies :
- Insulin Lispro Synthesis : Fmoc-Gly-Ser(Ψ(Me,Me)Pro)-OH enabled efficient assembly of the ester insulin intermediate, achieving a receptor-binding affinity (Kd = 0.12 ± 0.05 nM) comparable to native insulin . In contrast, stepwise SPPS without pseudoprolines resulted in <30% yield due to O/N-acyl shifts and transesterification .
- hGH-Derived Peptides : Substituting Gly with Arg in pseudoprolines (Fmoc-Arg(Pbf)-Ser(Ψ(Me,Me)Pro)-OH) was proposed to improve solubility in hydrophobic regions, but its unavailability necessitated alternative strategies .
Activité Biologique
Fmoc-Gly-Ser(Psi(Me,Me)Pro)-OH is a synthetic peptide derivative that has gained attention in biochemical and pharmaceutical research due to its unique structural features. The inclusion of the 9-fluorenylmethyloxycarbonyl (Fmoc) protective group, along with glycine (Gly), serine (Ser), and a pseudo-proline residue (Psi(Me,Me)Pro), enhances its stability and solubility. This article explores the biological activity of this compound, focusing on its synthesis, mechanism of action, applications in research, and comparative analysis with similar compounds.
Synthesis
The synthesis of this compound typically employs solid-phase peptide synthesis (SPPS). The process begins with the attachment of glycine to a solid support resin. The Fmoc group is then removed using a piperidine solution in dimethylformamide (DMF), allowing for the coupling of serine and the pseudo-proline residue using coupling reagents like N,N’-diisopropylcarbodiimide (DIC) and 1-hydroxybenzotriazole (HOBt) .
The presence of the pseudo-proline residue significantly influences the compound's biological activity by reducing intermolecular aggregation during peptide synthesis. This modification enhances solubility and coupling kinetics, making it a valuable tool for synthesizing larger peptides .
Biological Activity
The biological activity of this compound is primarily observed in its ability to facilitate peptide synthesis and influence protein interactions. Key aspects include:
- Stability : The incorporation of pseudo-proline residues reduces the likelihood of peptide aggregation, which is crucial for maintaining biological function .
- Enzyme Interactions : This compound can be utilized in studying enzyme-substrate interactions due to its structural properties that mimic natural substrates .
- Therapeutic Potential : Its role in developing peptide-based drugs highlights its significance in medicinal chemistry .
Comparative Analysis
To better understand the uniqueness of this compound, it can be compared with other related compounds:
Compound Name | Key Features | Stability | Applications |
---|---|---|---|
Fmoc-Gly-Ser-OH | Lacks pseudo-proline residue | Less stable | Limited applications |
Fmoc-Gly-Pro-OH | Contains proline instead of pseudo-proline | Moderate stability | Different chemical properties |
Fmoc-Gly-Ser(Psi(Me,Me)Ala)-OH | Contains pseudo-alanine | Varies | Similar applications |
This comparison illustrates how the inclusion of the pseudo-proline residue in this compound enhances its stability and versatility in various applications compared to other peptides.
Case Studies
Recent studies have utilized this compound in various contexts:
- Peptide Synthesis : Research demonstrated that using this compound significantly improved yields in synthesizing complex peptides due to enhanced solubility and reduced aggregation .
- Protein Interaction Studies : In a study examining protein-protein interactions, the incorporation of this peptide facilitated clearer results compared to traditional peptides lacking pseudo-proline modifications .
- Drug Development : Investigations into peptide-based therapeutics have shown that this compound can serve as a scaffold for designing novel drugs with improved pharmacological properties .
Q & A
Basic Research Questions
Q. How does Fmoc-Gly-Ser(Psi(Me,Me)Pro)-OH enhance coupling efficiency in solid-phase peptide synthesis (SPPS)?
this compound is a pseudoproline dipeptide monomer that disrupts secondary structures during SPPS, improving solubility and reducing aggregation. Studies show its acylation efficiency exceeds 96% even in challenging sequences, such as those with multiple arginine residues prone to δ-lactam formation. For example, in synthesizing the hGH-derived peptide, it facilitated coupling at positions 9 and 13, enabling high-purity crude products (42% purity) despite complex sequences .
Q. What are the optimal storage conditions for this compound to maintain stability?
The compound should be stored at -20°C in a dry environment. For long-term storage (up to 3 years), lyophilized powder is recommended. Solutions in DMSO should be aliquoted to avoid freeze-thaw cycles and stored at -80°C (6 months) or -20°C (1 month). Heating to 37°C and sonication can improve solubility if precipitation occurs .
Q. How can researchers verify the purity and quality of this compound batches?
Analytical methods include:
- HPLC with reverse-phase C18 columns (e.g., Agilent 1100 series) using gradients of acetonitrile/water with 0.1% TFA.
- LC-MS to confirm molecular weight (424.45 g/mol) and detect impurities.
- NMR (e.g., H-NMR) to validate structural integrity. Suppliers typically provide Certificates of Analysis (COA) with purity >98% .
Advanced Research Questions
Q. What strategies mitigate arginine isomerization during SPPS when using this compound?
Arginine δ-lactam formation can be minimized by:
- Using Fmoc-Arg(Pbf)-OH with slow coupling protocols to reduce side reactions.
- Incorporating pseudoproline residues (e.g., this compound) to disrupt β-sheet formation, which exacerbates isomerization.
- Employing in situ chemical ligation to avoid prolonged exposure of sensitive residues to basic conditions during Fmoc deprotection .
Q. What are the limitations of this compound in synthesizing peptides with multiple pseudoproline motifs?
While effective in improving solubility, overuse can lead to incomplete deprotection or steric hindrance. For example, in a 16-mer hGH peptide, introducing two pseudoproline residues (positions 12 and 13) resulted in complex product mixtures requiring MALDI-TOF for characterization. Additionally, sequences lacking Ser/Thr residues limit its applicability, and commercial availability of compatible dipeptides (e.g., Fmoc-Arg-Ser(Psi(Me,Me)Pro)-OH) remains a bottleneck .
Q. How does this compound compare to other pseudoproline dipeptides in preventing aggregation during SPPS?
Compared to Fmoc-Ala-Ser(Psi(Me,Me)Pro)-OH or Fmoc-Ile-Ser(Psi(Me,Me)Pro)-OH, the Gly variant offers greater flexibility due to its smaller side chain, reducing steric clashes. However, its efficacy depends on sequence context. For instance, in insulin lispro synthesis, ester bond instability (e.g., Glu[Oβ(Thr)]) required alternative strategies like native chemical ligation, where pseudoprolines alone were insufficient .
Q. Methodological Considerations
Q. What experimental adjustments are needed when using this compound in automated SPPS?
- Double coupling : Apply for residues following pseudoprolines to ensure complete acylation.
- Deprotection optimization : Use 20% piperidine in DMF with extended mixing times (5–10 minutes) to remove Fmoc groups without damaging the pseudoproline moiety.
- Side-chain protection : For cysteine-containing peptides, combine Trt and SIT (sec-isoamyl thiol) protections to minimize disulfide scrambling during cyclization .
Q. How can researchers troubleshoot low yields in pseudoproline-containing peptide cyclization?
Low cyclization efficiency often stems from residual aggregation or improper deprotection. Solutions include:
- Pre-cyclization purification : Use preparative HPLC to isolate linear peptides before oxidative folding.
- Solvent optimization : Employ high-dielectric solvents (e.g., 50% DMSO/water) to enhance solubility.
- Temperature control : Perform cyclization at 4°C to reduce side reactions .
Q. Data Contradictions and Validation
Q. How to resolve discrepancies between MALDI-TOF and LC-MS data for pseudoproline-containing peptides?
MALDI-TOF may fail to detect minor impurities due to ion suppression. Cross-validate with LC-MS/MS for fragmentation patterns. For example, in hGH peptide synthesis, MALDI-TOF identified the target mass, but LC-MS revealed byproducts from incomplete deprotection, necessitating gradient optimization .
Q. Why do some studies report >95% coupling efficiency for this compound, while others observe aggregation?
Efficiency depends on sequence context. In hydrophobic or β-sheet-prone regions (e.g., Val/Leu-rich motifs), even pseudoprolines may fail to prevent aggregation. Pre-activating the residue with HOBt/DIC or switching to ChemMatrix resin (higher swelling capacity) can improve results .
Propriétés
IUPAC Name |
(4S)-3-[2-(9H-fluoren-9-ylmethoxycarbonylamino)acetyl]-2,2-dimethyl-1,3-oxazolidine-4-carboxylic acid | |
---|---|---|
Source | PubChem | |
URL | https://pubchem.ncbi.nlm.nih.gov | |
Description | Data deposited in or computed by PubChem | |
InChI |
InChI=1S/C23H24N2O6/c1-23(2)25(19(13-31-23)21(27)28)20(26)11-24-22(29)30-12-18-16-9-5-3-7-14(16)15-8-4-6-10-17(15)18/h3-10,18-19H,11-13H2,1-2H3,(H,24,29)(H,27,28)/t19-/m0/s1 | |
Source | PubChem | |
URL | https://pubchem.ncbi.nlm.nih.gov | |
Description | Data deposited in or computed by PubChem | |
InChI Key |
XUBXHZJERGBCEL-IBGZPJMESA-N | |
Source | PubChem | |
URL | https://pubchem.ncbi.nlm.nih.gov | |
Description | Data deposited in or computed by PubChem | |
Canonical SMILES |
CC1(N(C(CO1)C(=O)O)C(=O)CNC(=O)OCC2C3=CC=CC=C3C4=CC=CC=C24)C | |
Source | PubChem | |
URL | https://pubchem.ncbi.nlm.nih.gov | |
Description | Data deposited in or computed by PubChem | |
Isomeric SMILES |
CC1(N([C@@H](CO1)C(=O)O)C(=O)CNC(=O)OCC2C3=CC=CC=C3C4=CC=CC=C24)C | |
Source | PubChem | |
URL | https://pubchem.ncbi.nlm.nih.gov | |
Description | Data deposited in or computed by PubChem | |
Molecular Formula |
C23H24N2O6 | |
Source | PubChem | |
URL | https://pubchem.ncbi.nlm.nih.gov | |
Description | Data deposited in or computed by PubChem | |
Molecular Weight |
424.4 g/mol | |
Source | PubChem | |
URL | https://pubchem.ncbi.nlm.nih.gov | |
Description | Data deposited in or computed by PubChem | |
Avertissement et informations sur les produits de recherche in vitro
Veuillez noter que tous les articles et informations sur les produits présentés sur BenchChem sont destinés uniquement à des fins informatives. Les produits disponibles à l'achat sur BenchChem sont spécifiquement conçus pour des études in vitro, qui sont réalisées en dehors des organismes vivants. Les études in vitro, dérivées du terme latin "in verre", impliquent des expériences réalisées dans des environnements de laboratoire contrôlés à l'aide de cellules ou de tissus. Il est important de noter que ces produits ne sont pas classés comme médicaments et n'ont pas reçu l'approbation de la FDA pour la prévention, le traitement ou la guérison de toute condition médicale, affection ou maladie. Nous devons souligner que toute forme d'introduction corporelle de ces produits chez les humains ou les animaux est strictement interdite par la loi. Il est essentiel de respecter ces directives pour assurer la conformité aux normes légales et éthiques en matière de recherche et d'expérimentation.