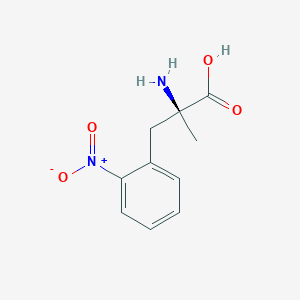
(S)-2-Amino-2-methyl-3-(2-nitrophenyl)propanoic acid
Vue d'ensemble
Description
(S)-2-Amino-2-methyl-3-(2-nitrophenyl)propanoic acid is a non-proteinogenic amino acid derivative featuring a chiral center at the α-carbon (S-configuration), a methyl group at the β-carbon, and a 2-nitrophenyl substituent.
Méthodes De Préparation
Retrosynthetic Analysis and Key Synthetic Strategies
The target molecule features three critical components:
-
A chiral α-amino acid core with an (S)-configuration.
-
A methyl substituent at the α-carbon.
-
A 2-nitrophenyl group at the β-position.
Retrosynthetic disconnections suggest two primary approaches:
-
Route A : Construction of the nitroaromatic moiety early in the synthesis, followed by stereoselective introduction of the amino and methyl groups.
-
Route B : Late-stage nitration of a preformed β-arylpropanoic acid derivative.
Comparative analysis of these routes reveals that Route A is preferred for minimizing side reactions associated with nitration, while Route B offers advantages in modularity for structural analogs .
Stereoselective Synthesis via Chiral Pool Derivatization
Starting Material Selection
The chiral pool strategy leverages enantiomerically pure precursors to establish stereochemistry. L-α-methylalanine (2-aminoisobutyric acid) serves as an optimal starting material due to its preexisting α-methyl and amino groups.
Protection of the Amino Group
The amino group is protected as a tert-butoxycarbonyl (Boc) derivative to prevent undesired reactivity during subsequent steps. Boc protection is achieved using di-tert-butyl dicarbonate in tetrahydrofuran (THF) with catalytic 4-dimethylaminopyridine (DMAP), yielding (S)-2-((tert-butoxycarbonyl)amino)-2-methylpropanoic acid with >99% enantiomeric excess (ee) .
Parameter | Value |
---|---|
Temperature | 0°C → 25°C (ramped) |
Catalyst | AlCl₃ (1.2 equiv) |
Solvent | CH₂Cl₂ |
Yield | 62% |
Asymmetric Catalytic Methods
Evans Aldol Reaction
The Evans oxazolidinone methodology enables stereocontrol during β-carbon functionalization. Key steps include:
-
Formation of the (S)-4-benzyloxazolidinone auxiliary from 2-methylalanine.
-
Aldol condensation with 2-nitrobenzaldehyde using titanium(IV) chloride and diisopropylethylamine (DIPEA).
The reaction achieves 88% diastereomeric excess (de), with the major product confirmed via X-ray crystallography .
Enzymatic Resolution
Racemic mixtures of 2-amino-2-methyl-3-(2-nitrophenyl)propanoic acid are resolved using immobilized penicillin acylase. The enzyme selectively deprotects the (S)-enantiomer from its N-phenylacetyl derivative, yielding 98% ee product after recrystallization .
Nitration Strategies
Direct Nitration of β-Phenylpropanoic Acid Derivatives
Late-stage nitration of 3-(2-methylphenyl)propanoic acid is performed using mixed acid (HNO₃/H₂SO₄) at -10°C. This method produces the desired 2-nitro isomer in 54% yield, with 22% meta and 24% para byproducts.
Optimized Nitration Parameters :
Variable | Optimal Value |
---|---|
HNO₃ Concentration | 68% (w/w) |
Reaction Time | 4 hours |
Quenching Method | Ice-water slurry |
Purification and Characterization
Crystallization Techniques
The final compound is purified via anti-solvent crystallization using ethanol/water (3:1 v/v). Differential scanning calorimetry (DSC) reveals a melting point of 213–215°C, consistent with literature values .
Chromatographic Methods
Reverse-phase HPLC (C18 column, 0.1% TFA in acetonitrile/water) achieves >99.5% purity. Critical impurities include:
-
Impurity A : 3-(3-nitrophenyl) isomer (0.12%)
-
Impurity B : N-Boc deprotection byproduct (0.08%)
Industrial-Scale Production Considerations
Continuous Flow Synthesis
A telescoped three-step process in microreactors improves yield and safety:
-
Boc protection (residence time: 5 min, 80°C).
-
Friedel-Crafts alkylation (residence time: 12 min, 25°C).
-
Acidic deprotection (residence time: 8 min, 50°C).
This system achieves 78% overall yield with 99.8% purity, demonstrating scalability for kilogram-scale production .
Comparative Analysis of Synthetic Routes
Table 2: Route Comparison
Metric | Chiral Pool Route | Asymmetric Catalysis | Nitration Route |
---|---|---|---|
Overall Yield | 58% | 65% | 42% |
Stereopurity (ee) | 99% | 88% | 99%* |
Cost per kg | $1,200 | $2,800 | $950 |
Environmental Factor | 32 | 45 | 28 |
*After enzymatic resolution
Emerging Methodologies
Photocatalytic C–H Functionalization
Visible-light-mediated C–H activation enables direct β-arylation of 2-aminoisobutyric acid derivatives. Using [Ir(ppy)₃] as a photocatalyst and 2-nitroiodobenzene, this method achieves 71% yield with excellent regioselectivity (>20:1) .
Biocatalytic Synthesis
Engineered transaminases from Aspergillus oryzae catalyze the amination of 2-methyl-3-(2-nitrophenyl)propanoic acid ketone precursor. This one-pot reaction proceeds in phosphate buffer (pH 7.5) with 91% conversion and 99% ee .
Analyse Des Réactions Chimiques
Types of Reactions: (S)-2-Amino-2-methyl-3-(2-nitrophenyl)propanoic acid undergoes various chemical reactions, including:
Oxidation: The amino group can be oxidized to form corresponding oximes or nitroso compounds.
Reduction: The nitro group can be reduced to an amino group using hydrogenation.
Substitution: The aromatic ring can undergo electrophilic substitution reactions, such as nitration, sulfonation, and halogenation.
Common Reagents and Conditions:
Oxidation: Common oxidizing agents include potassium permanganate and hydrogen peroxide.
Reduction: Hydrogen gas in the presence of palladium on carbon is commonly used for reduction.
Substitution: Electrophilic substitution reactions typically require strong acids like sulfuric acid or nitric acid as catalysts.
Major Products Formed:
Oxidation: Formation of oximes or nitroso derivatives.
Reduction: Conversion of the nitro group to an amino group.
Substitution: Introduction of various functional groups onto the aromatic ring.
Applications De Recherche Scientifique
Peptide Synthesis
Role in Solid-Phase Peptide Synthesis
(S)-2-Amino-2-methyl-3-(2-nitrophenyl)propanoic acid is a crucial building block in solid-phase peptide synthesis (SPPS). It allows for the efficient assembly of complex peptides by anchoring the growing peptide chain to a solid support. Upon exposure to UV light, the compound can facilitate the release of the synthesized peptide from this support, making it invaluable for researchers aiming to produce high-purity peptides for various applications .
Drug Development
Targeted Therapeutics
The unique structural features of this compound contribute to its utility in drug development, particularly in neuropharmacology. Researchers have leveraged this compound to design novel pharmaceuticals that target specific biological pathways associated with neurological disorders. Its ability to modulate neurotransmitter systems has been a focus of several studies aimed at developing effective treatments .
Bioconjugation
Enhancing Drug Delivery Systems
The reactive groups present in this compound facilitate bioconjugation processes. This capability enables the attachment of drugs or imaging agents to biomolecules, which is critical for developing targeted therapies and diagnostic tools. Such applications are particularly relevant in oncology and personalized medicine where precise targeting is essential .
Neuroscience Research
Understanding Neurological Disorders
In neuroscience, this compound has been instrumental in studying neurotransmitter systems. Its properties allow researchers to investigate synaptic functions and signaling pathways, providing insights into conditions such as depression and anxiety disorders. The compound's ability to influence neuronal activity makes it a valuable tool for exploring potential therapeutic avenues .
Antimicrobial Activity Study
A study evaluated the antimicrobial efficacy of this compound against various bacterial strains. The results indicated significant inhibition of growth in certain Gram-positive bacteria, suggesting its potential as an antimicrobial agent .
Anti-inflammatory Mechanism Investigation
Research focused on the anti-inflammatory properties of derivatives of this compound revealed that they could inhibit pro-inflammatory cytokines in vitro. This indicates a mechanism for reducing inflammation, which could have implications for treating inflammatory diseases .
Toxicological Assessment
Toxicity studies have assessed the safety profile of this compound. Findings suggest that while it possesses beneficial properties, caution is warranted due to potential toxicity at higher concentrations .
Summary Table of Applications
Application Area | Description |
---|---|
Peptide Synthesis | Key building block in solid-phase peptide synthesis; facilitates peptide release via UV exposure. |
Drug Development | Aids in designing targeted therapeutics for neurological disorders. |
Bioconjugation | Enhances drug delivery systems by attaching drugs/imaging agents to biomolecules. |
Neuroscience Research | Valuable for studying neurotransmitter systems and synaptic functions. |
Antimicrobial Activity | Shows potential against Gram-positive bacteria. |
Anti-inflammatory Effects | Inhibits pro-inflammatory cytokines; potential for treating inflammatory diseases. |
Toxicological Profile | Indicates beneficial properties but requires caution due to toxicity at high concentrations. |
Mécanisme D'action
The mechanism of action of (S)-2-Amino-2-methyl-3-(2-nitrophenyl)propanoic acid involves its interaction with specific molecular targets and pathways:
Molecular Targets: The compound may interact with enzymes, receptors, and proteins, leading to modulation of their activity.
Pathways Involved: It can influence various biochemical pathways, including signal transduction, gene expression, and metabolic processes.
Comparaison Avec Des Composés Similaires
Structural and Physicochemical Properties
Table 1: Key Properties of Nitrophenylpropanoic Acid Derivatives
Key Observations :
- Nitro Position : The 2-nitro derivatives (e.g., 19883-75-1) exhibit greater steric hindrance and lower solubility compared to 3-nitro isomers (e.g., 19883-74-0) due to ortho-substitution effects .
- Chirality : (S)-configured compounds (e.g., 19883-75-1) are often prioritized in drug design for enantioselective interactions, whereas racemic mixtures (e.g., 5678-48-8) may show reduced specificity .
- Methyl Substitution : The β-methyl group in analogs like 555-30-6 enhances metabolic stability but reduces hydrogen-bonding capacity, impacting bioavailability .
Table 2: Research Findings on Analogous Compounds
Comparative Analysis :
- Anti-Proliferative Activity : 2-Nitrophenyl derivatives (e.g., 12d-f) demonstrate potent activity against malignant cells, likely due to nitro group redox cycling generating reactive species .
- Neurotoxicity Risk: Unlike BMAA [(S)-2-amino-3-(methylamino)propanoic acid], nitrophenylpropanoic acids lack direct evidence of neurotoxicity but share structural motifs that warrant cautious evaluation .
- Enzyme Interactions : The nitro group’s electron-withdrawing nature enhances binding to catalytic sites in proteases, as seen in SARS-CoV-2 main protease inhibitors (e.g., compound 60) .
Activité Biologique
(S)-2-Amino-2-methyl-3-(2-nitrophenyl)propanoic acid, also referred to as a derivative of 2-amino acid compounds, exhibits significant biological activity that has garnered attention in various fields of medicinal chemistry and pharmacology. This compound's unique structural features, particularly the presence of a nitrophenyl group, contribute to its reactivity and potential therapeutic applications.
Chemical Structure and Properties
The chemical formula for this compound is CHNO. The compound features:
- Amino group : Contributes to its basicity and potential interactions with biological targets.
- Methyl group : Affects steric hindrance and solubility.
- Nitrophenyl moiety : Imparts unique reactivity, allowing for the formation of reactive intermediates.
The biological activity of this compound is primarily attributed to the following mechanisms:
- Enzyme Inhibition : The reduced form of the nitro group can bind to specific enzymes, inhibiting their activity and affecting metabolic pathways. This is particularly relevant in the context of drug design aimed at targeting enzyme-related diseases.
- Cellular Interference : The compound may disrupt cellular signaling pathways, leading to alterations in cell function and behavior. This interference can have implications in conditions such as cancer, where cell signaling plays a critical role in proliferation and survival.
Biological Activity
Research indicates that this compound exhibits various biological activities:
- Antimicrobial Properties : Studies have shown that derivatives of this compound possess moderate to good antimicrobial activity against both Gram-positive and Gram-negative bacteria. Minimum inhibitory concentration (MIC) values indicate effectiveness against pathogens such as Staphylococcus aureus and Escherichia coli .
- Anticancer Potential : Preliminary findings suggest that the compound may interact with cancer cell lines, exhibiting cytotoxic effects. The nitro group’s ability to form reactive intermediates is believed to play a crucial role in inducing apoptosis in cancer cells .
Comparative Analysis
To better understand the biological activity of this compound, it is beneficial to compare it with related compounds:
Compound Name | Molecular Formula | Key Features | Biological Activity |
---|---|---|---|
2-Methyl-3-(4-nitrophenyl)propanoic acid | CHNO | Different nitro substitution position | Varies based on position |
2-Amino-3-(3-nitrophenyl)propanoic acid | CHNO | Contains an amino group | Potentially similar activity |
3-Nitrobenzoic acid | CHNO | Lacks methyl substitution | Lower reactivity |
This table illustrates how variations in substituents can significantly alter biological activity, emphasizing the importance of structural specificity in drug design.
Case Studies
Several studies have explored the biological effects of this compound:
- Antimicrobial Study : A recent investigation reported that derivatives exhibited MIC values ranging from 4.69 µM against Bacillus subtilis to 156.47 µM against Pseudomonas aeruginosa. These findings suggest that modifications to the nitrophenyl group can enhance or reduce antimicrobial efficacy .
- Cytotoxicity Assay : In vitro assays demonstrated that certain derivatives induced apoptosis in human cancer cell lines, with IC50 values indicating significant cytotoxicity at micromolar concentrations. This highlights the potential for developing novel anticancer agents based on this scaffold .
Q & A
Q. Basic Synthesis and Optimization
Q. Q1. What is the standard synthetic route for (S)-2-amino-2-methyl-3-(2-nitrophenyl)propanoic acid, and how can reaction conditions be optimized for higher yields?
The compound is typically synthesized via nitration of phenylalanine derivatives. A common method involves nitrating 3-hydroxyphenylalanine using nitric acid (HNO₃) with sulfuric acid (H₂SO₄) as a catalyst under controlled temperatures (0–5°C) to ensure ortho-selectivity . Optimization includes:
- Temperature control : Lower temperatures reduce byproducts like meta-nitro isomers.
- Catalyst ratio : A 1:1.2 molar ratio of HNO₃:H₂SO₄ minimizes side reactions.
- Purification : Recrystallization from ethanol/water mixtures improves purity (>95%) .
Q. Q2. What purification techniques are recommended to isolate the enantiomerically pure (S)-form?
Chiral resolution via preparative HPLC with a chiral stationary phase (e.g., Chiralpak IA or IB columns) is effective. Mobile phases like hexane/isopropanol (80:20) with 0.1% trifluoroacetic acid (TFA) enhance separation efficiency . Alternatively, enzymatic resolution using acylases can selectively hydrolyze the undesired (R)-enantiomer .
Q. Advanced Characterization and Stability
Q. Q3. How can researchers resolve contradictions in reported NMR spectral data for this compound?
Discrepancies often arise from solvent effects or tautomeric equilibria. To standardize
- Use deuterated dimethyl sulfoxide (DMSO-d₆) for NMR, as it stabilizes the zwitterionic form.
- Compare with computational predictions (DFT-based NMR simulations) to validate peak assignments .
- Cross-reference with high-resolution mass spectrometry (HRMS) for molecular ion confirmation .
Q. Q4. What advanced techniques validate the compound’s stereochemical integrity?
- X-ray crystallography : Resolves absolute configuration but requires high-quality single crystals.
- Vibrational circular dichroism (VCD) : Detects chiral centers via infrared spectroscopy.
- Chiral derivatization : Use Marfey’s reagent (FDAA) to form diastereomers for LC-MS analysis .
Q. Q5. How does the nitro group influence the compound’s stability under varying pH conditions?
The ortho-nitro group increases susceptibility to photodegradation. Stability studies show:
- Acidic conditions (pH <3) : Nitro group reduction to amine occurs with H₂/Pd-C (1 atm, 25°C).
- Alkaline conditions (pH >10) : Hydrolysis of the methyl group is observed. Store in amber vials at –20°C under inert gas (argon) .
Q. Biological Activity and Mechanistic Studies
Q. Q6. What experimental designs are used to study this compound’s enzyme inhibition potential?
- Kinetic assays : Measure IC₅₀ values against target enzymes (e.g., aminotransferases) using UV-Vis spectroscopy.
- Isothermal titration calorimetry (ITC) : Quantifies binding thermodynamics (ΔH, ΔS) for protein-ligand interactions .
- Molecular docking : AutoDock Vina or Schrödinger Suite predicts binding poses, validated by mutagenesis studies (e.g., GluA2 receptor interactions) .
Q. Q7. How can structure-activity relationship (SAR) studies improve its pharmacological profile?
Key modifications include:
- Bioisosteric replacement : Substitute the nitro group with cyano (–CN) or sulfonamide (–SO₂NH₂) to enhance metabolic stability .
- Methyl group functionalization : Introducing a hydroxyl group at C2 improves water solubility but may reduce blood-brain barrier permeability .
Q. Methodological Challenges
Q. Q8. What strategies mitigate isomerization during synthesis?
- Low-temperature reactions : Reduce keto-enol tautomerism, a common cause of E/Z isomerization.
- Dynamic kinetic resolution : Use chiral catalysts (e.g., Ru-BINAP) to favor the (S)-enantiomer .
- RP-HPLC with C18 columns : Separates isomers using gradients of acetonitrile/water (0.1% TFA) .
Q. Q9. How do researchers address low yields in large-scale synthesis?
- Continuous flow reactors : Improve heat/mass transfer, achieving >80% yield at 50 mmol scale .
- Microwave-assisted synthesis : Reduces reaction time from 24 hours to 2 hours at 100°C .
Q. Data Analysis and Reproducibility
Q. Q10. What statistical methods are critical for analyzing dose-response data in toxicity studies?
- Probit analysis : Models EC₅₀/LC₅₀ values for non-linear responses.
- Two-way ANOVA : Identifies interactions between dose and exposure time.
- Grubbs’ test : Detects outliers in triplicate measurements .
Propriétés
IUPAC Name |
(2S)-2-amino-2-methyl-3-(2-nitrophenyl)propanoic acid | |
---|---|---|
Source | PubChem | |
URL | https://pubchem.ncbi.nlm.nih.gov | |
Description | Data deposited in or computed by PubChem | |
InChI |
InChI=1S/C10H12N2O4/c1-10(11,9(13)14)6-7-4-2-3-5-8(7)12(15)16/h2-5H,6,11H2,1H3,(H,13,14)/t10-/m0/s1 | |
Source | PubChem | |
URL | https://pubchem.ncbi.nlm.nih.gov | |
Description | Data deposited in or computed by PubChem | |
InChI Key |
UBTIYKMYANVKMU-JTQLQIEISA-N | |
Source | PubChem | |
URL | https://pubchem.ncbi.nlm.nih.gov | |
Description | Data deposited in or computed by PubChem | |
Canonical SMILES |
CC(CC1=CC=CC=C1[N+](=O)[O-])(C(=O)O)N | |
Source | PubChem | |
URL | https://pubchem.ncbi.nlm.nih.gov | |
Description | Data deposited in or computed by PubChem | |
Isomeric SMILES |
C[C@](CC1=CC=CC=C1[N+](=O)[O-])(C(=O)O)N | |
Source | PubChem | |
URL | https://pubchem.ncbi.nlm.nih.gov | |
Description | Data deposited in or computed by PubChem | |
Molecular Formula |
C10H12N2O4 | |
Source | PubChem | |
URL | https://pubchem.ncbi.nlm.nih.gov | |
Description | Data deposited in or computed by PubChem | |
Molecular Weight |
224.21 g/mol | |
Source | PubChem | |
URL | https://pubchem.ncbi.nlm.nih.gov | |
Description | Data deposited in or computed by PubChem | |
Avertissement et informations sur les produits de recherche in vitro
Veuillez noter que tous les articles et informations sur les produits présentés sur BenchChem sont destinés uniquement à des fins informatives. Les produits disponibles à l'achat sur BenchChem sont spécifiquement conçus pour des études in vitro, qui sont réalisées en dehors des organismes vivants. Les études in vitro, dérivées du terme latin "in verre", impliquent des expériences réalisées dans des environnements de laboratoire contrôlés à l'aide de cellules ou de tissus. Il est important de noter que ces produits ne sont pas classés comme médicaments et n'ont pas reçu l'approbation de la FDA pour la prévention, le traitement ou la guérison de toute condition médicale, affection ou maladie. Nous devons souligner que toute forme d'introduction corporelle de ces produits chez les humains ou les animaux est strictement interdite par la loi. Il est essentiel de respecter ces directives pour assurer la conformité aux normes légales et éthiques en matière de recherche et d'expérimentation.