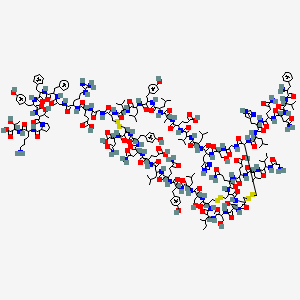
Recombinant insulin Human
- Cliquez sur DEMANDE RAPIDE pour recevoir un devis de notre équipe d'experts.
- Avec des produits de qualité à un prix COMPÉTITIF, vous pouvez vous concentrer davantage sur votre recherche.
Vue d'ensemble
Description
Insuline recombinante: est une forme synthétique d'insuline humaine produite par génie génétique. Cette technologie permet d'insérer le gène de l'insuline humaine dans le matériel génétique d'une bactérie courante, permettant à la bactérie de produire de l'insuline humaine . L'insuline recombinante est identique à l'insuline naturellement produite par le pancréas et est utilisée pour gérer les niveaux de glucose sanguin chez les personnes atteintes de diabète sucré .
Applications De Recherche Scientifique
Chemistry: Insulin recombinant serves as a model protein for studying protein folding, disulfide bond formation, and recombinant protein production techniques .
Biology: In biological research, insulin recombinant is used to study insulin signaling pathways, glucose metabolism, and the regulation of blood sugar levels .
Medicine: The primary application of insulin recombinant is in the treatment of diabetes mellitus. It is used to manage blood glucose levels in individuals with type 1 and type 2 diabetes . Insulin analogs, which are modified forms of insulin recombinant, have been developed to provide different pharmacokinetic profiles for better glycemic control .
Industry: In the pharmaceutical industry, insulin recombinant is produced on a large scale to meet the global demand for insulin therapy .
Mécanisme D'action
Target of Action
Recombinant insulin human, also known as regular insulin, is a short-acting form of insulin used for the treatment of hyperglycemia caused by Type 1 and Type 2 Diabetes . It is produced by recombinant DNA technology and is identical to endogenously produced insulin . The primary targets of insulin are the liver, fat cells, and skeletal muscle . Insulin is a peptide hormone produced by beta cells of the pancreas that promotes glucose metabolism .
Mode of Action
Insulin binds with specific receptors on the outer membrane of target cells, which causes metabolic processes to take place within those cells . Insulin is released from the pancreas following a meal to promote the uptake of glucose from the blood into internal organs and tissues such as the liver, fat cells, and skeletal muscle . Absorption of glucose into cells allows for its transformation into glycogen or fat for storage . Insulin also inhibits hepatic glucose production, enhances protein synthesis, and inhibits lipolysis and proteolysis among many other functions .
Biochemical Pathways
The biochemical pathways affected by insulin involve the uptake of glucose by cells and its transformation into glycogen or fat for storage . Insulin also inhibits hepatic glucose production, enhances protein synthesis, and inhibits lipolysis and proteolysis . These processes are crucial for maintaining glucose homeostasis in the body.
Pharmacokinetics
The pharmacokinetics of insulin involve its absorption, distribution, metabolism, and excretion (ADME). After administration, insulin is absorbed into the bloodstream and distributed to various tissues, primarily muscle and fat, where it imparts a variety of biochemical processes . The metabolism and excretion of insulin are complex processes involving the liver and kidneys .
Result of Action
The result of insulin’s action is the regulation of glucose metabolism. By promoting the uptake of glucose from the blood into internal organs and tissues, insulin helps to lower blood glucose levels . This is crucial in the management of diabetes mellitus, a condition characterized by high blood glucose levels .
Action Environment
The action of insulin can be influenced by various environmental factors. For instance, the production of recombinant insulin in E. coli requires a massive investment in bioreactors . Moreover, the efficacy and stability of insulin can be affected by factors such as temperature and pH . Therefore, careful control of the production environment is necessary to ensure the quality and effectiveness of recombinant insulin.
Orientations Futures
The demand for recombinant insulin is expected to increase due to the rising prevalence of diabetes worldwide . There is a pressing need to develop a more efficient and economical production process . Future developments in therapeutic peptides, genome engineering, and editing technologies for adequate insulin and insulin analogs production are being explored .
Analyse Biochimique
Biochemical Properties
Recombinant Insulin Human plays a crucial role in biochemical reactions. It interacts with the insulin receptor, a protein on the surface of cells . Upon binding, it triggers a series of reactions within the cell that allows glucose to be taken up from the bloodstream .
Cellular Effects
This compound has profound effects on various types of cells and cellular processes. It influences cell function by impacting cell signaling pathways, gene expression, and cellular metabolism . For instance, it stimulates the uptake of glucose in muscle and fat cells and inhibits the production of glucose in the liver .
Molecular Mechanism
The mechanism of action of this compound involves binding to the insulin receptor on the cell surface . This binding activates the receptor, leading to its autophosphorylation . This, in turn, triggers a cascade of intracellular events, including the translocation of glucose transporters to the cell membrane and the uptake of glucose .
Temporal Effects in Laboratory Settings
In laboratory settings, the effects of this compound can change over time. Studies have shown that it remains stable under various conditions, and its effects on cellular function have been observed in both in vitro and in vivo studies .
Dosage Effects in Animal Models
The effects of this compound can vary with different dosages in animal models. While low doses can effectively lower blood glucose levels, high doses may lead to hypoglycemia, a condition characterized by abnormally low blood glucose levels .
Metabolic Pathways
This compound is involved in several metabolic pathways. It interacts with enzymes and cofactors in the glycolysis pathway, the tricarboxylic acid cycle, and the pentose phosphate pathway . It can also affect metabolic flux and metabolite levels .
Transport and Distribution
This compound is transported and distributed within cells and tissues through various mechanisms. It can bind to insulin receptors on the cell surface, which can trigger its internalization and subsequent distribution within the cell .
Subcellular Localization
The subcellular localization of this compound can affect its activity or function. Once inside the cell, it can be directed to specific compartments or organelles based on targeting signals or post-translational modifications .
Méthodes De Préparation
Voies de synthèse et conditions de réaction: La production d'insuline recombinante implique plusieurs étapes clés :
Insertion du gène: Le gène de l'insuline humaine est inséré dans un plasmide, qui est ensuite introduit dans une bactérie telle qu'Escherichia coli.
Fermentation: Les bactéries recombinantes sont cultivées dans de grands réservoirs de fermentation, où elles produisent de la pro-insuline.
Récupération de la pro-insuline: La pro-insuline est récupérée à partir des corps d'inclusion au sein des bactéries.
Solubilisation et clivage: Les corps d'inclusion sont solubilisés et la pro-insuline est clivée pour former de l'insuline.
Purification: L'insuline est purifiée par chromatographie et autres techniques pour garantir sa pureté et son efficacité.
Méthodes de production industrielle: La production industrielle d'insuline recombinante implique généralement des procédés de fermentation à grande échelle utilisant des cellules d'Escherichia coli ou de levure génétiquement modifiées. Le processus comprend :
Fermentation: De grands bioréacteurs sont utilisés pour cultiver les micro-organismes recombinants.
Récolte: Les cellules sont récoltées et la pro-insuline est extraite.
Purification: La pro-insuline subit plusieurs étapes de purification, notamment la chromatographie, pour éliminer les impuretés.
Formulation: L'insuline purifiée est formulée sous une forme stable et injectable.
Analyse Des Réactions Chimiques
Types de réactions: L'insuline recombinante subit plusieurs réactions chimiques pendant sa synthèse et sa purification :
Réactifs et conditions courants :
Sulfitolyse oxydative: Utilisée pour solubiliser les corps d'inclusion et faciliter la formation de liaisons disulfure correctes.
Bromure de cyanogène: Utilisé pour le clivage de la pro-insuline.
Tampons de chromatographie: Différents tampons sont utilisés pendant le processus de purification pour maintenir la stabilité et l'activité de l'insuline.
Principaux produits: Le principal produit de ces réactions est l'insuline recombinante, qui est identique à l'insuline humaine en structure et en fonction .
Applications de recherche scientifique
Chimie: L'insuline recombinante sert de protéine modèle pour étudier le repliement des protéines, la formation de liaisons disulfure et les techniques de production de protéines recombinantes .
Biologie: En recherche biologique, l'insuline recombinante est utilisée pour étudier les voies de signalisation de l'insuline, le métabolisme du glucose et la régulation des niveaux de sucre dans le sang .
Médecine: La principale application de l'insuline recombinante est le traitement du diabète sucré. Elle est utilisée pour gérer les niveaux de glucose sanguin chez les personnes atteintes de diabète de type 1 et de type 2 . Des analogues de l'insuline, qui sont des formes modifiées de l'insuline recombinante, ont été développés pour fournir différents profils pharmacocinétiques pour une meilleure maîtrise glycémique .
Industrie: Dans l'industrie pharmaceutique, l'insuline recombinante est produite à grande échelle pour répondre à la demande mondiale en insulinothérapie .
Mécanisme d'action
L'insuline recombinante exerce ses effets en se liant aux récepteurs de l'insuline à la surface des cellules cibles, telles que les cellules du foie, des muscles et des graisses . Cette liaison active le récepteur de l'insuline, ce qui déclenche une cascade de voies de signalisation intracellulaires qui favorisent l'absorption du glucose, la synthèse du glycogène et l'inhibition de la néoglucogenèse . Les principales cibles moléculaires comprennent le récepteur de l'insuline et les molécules de signalisation en aval telles que les protéines du substrat du récepteur de l'insuline (IRS) et la phosphoinositide 3-kinase (PI3K) .
Comparaison Avec Des Composés Similaires
Composés similaires :
Insuline aspart: Un autre analogue de l'insuline à action rapide avec une seule substitution d'acide aminé pour améliorer l'absorption.
Insuline glargine: Un analogue de l'insuline à action prolongée conçu pour fournir une libération régulière d'insuline sur 24 heures.
Unicité: L'insuline recombinante est unique en ce qu'elle est identique à l'insuline humaine, ce qui la rend très efficace et bien tolérée chez les patients. Contrairement aux analogues de l'insuline, qui ont des structures modifiées pour modifier leurs propriétés pharmacocinétiques, l'insuline recombinante imite l'insuline naturelle produite par le pancréas .
Propriétés
{ "Design of the Synthesis Pathway": "The synthesis pathway for recombinant insulin Human involves the use of genetic engineering techniques to produce the insulin protein in a bacterial host cell. The gene for human insulin is inserted into the bacterial genome, and the host cell is then induced to produce the insulin protein. The protein is then purified and processed to produce the final recombinant insulin Human product.", "Starting Materials": [ "Bacterial host cell (e.g. Escherichia coli)", "Gene for human insulin", "Culture media for bacterial growth", "Purification reagents" ], "Reaction": [ "Insertion of human insulin gene into bacterial genome using recombinant DNA technology", "Culturing of transformed bacterial cells in culture media to induce insulin protein production", "Harvesting of insulin protein from bacterial cells", "Purification of insulin protein using chromatography techniques", "Processing of purified insulin protein to produce final recombinant insulin Human product" ] } | |
Numéro CAS |
11061-68-0 |
Formule moléculaire |
C257H383N65O77S6 |
Poids moléculaire |
5808 g/mol |
Nom IUPAC |
(4S)-4-[[2-[[(1R,6R,12S,15S,18S,21S,24S,27S,30S,33S,36S,39S,42R,47R,50S,53S,56S,59S,62S,65S,68S,71S,74R,77S,80S,83S,88R)-88-[[(2S)-5-amino-2-[[(2S)-2-[[(2S)-2-[[(2S,3S)-2-[(2-aminoacetyl)amino]-3-methylpentanoyl]amino]-3-methylbutanoyl]amino]-4-carboxybutanoyl]amino]-5-oxopentanoyl]amino]-6-[[(2S)-2-[[(2S)-2-[[(2S)-5-amino-2-[[(2S)-4-amino-2-[[(2S)-2-[[(2S)-2-amino-3-phenylpropanoyl]amino]-3-methylbutanoyl]amino]-4-oxobutanoyl]amino]-5-oxopentanoyl]amino]-3-(1H-imidazol-4-yl)propanoyl]amino]-4-methylpentanoyl]amino]-47-[[(1S)-3-amino-1-carboxy-3-oxopropyl]carbamoyl]-53-(2-amino-2-oxoethyl)-62-(3-amino-3-oxopropyl)-77-[(2S)-butan-2-yl]-24,56-bis(2-carboxyethyl)-83-[(1R)-1-hydroxyethyl]-12,71,80-tris(hydroxymethyl)-33,50,65-tris[(4-hydroxyphenyl)methyl]-15-(1H-imidazol-4-ylmethyl)-27-methyl-18,30,36,59,68-pentakis(2-methylpropyl)-7,10,13,16,19,22,25,28,31,34,37,40,49,52,55,58,61,64,67,70,73,76,79,82,85,87-hexacosaoxo-21,39-di(propan-2-yl)-3,4,44,45,90,91-hexathia-8,11,14,17,20,23,26,29,32,35,38,41,48,51,54,57,60,63,66,69,72,75,78,81,84,86-hexacosazabicyclo[72.11.7]dononacontane-42-carbonyl]amino]acetyl]amino]-5-[[(2S)-1-[[2-[[(2S)-1-[[(2S)-1-[[(2S)-1-[[(2S,3R)-1-[(2S)-2-[[(2S)-6-amino-1-[[(1S,2R)-1-carboxy-2-hydroxypropyl]amino]-1-oxohexan-2-yl]carbamoyl]pyrrolidin-1-yl]-3-hydroxy-1-oxobutan-2-yl]amino]-3-(4-hydroxyphenyl)-1-oxopropan-2-yl]amino]-1-oxo-3-phenylpropan-2-yl]amino]-1-oxo-3-phenylpropan-2-yl]amino]-2-oxoethyl]amino]-5-carbamimidamido-1-oxopentan-2-yl]amino]-5-oxopentanoic acid |
InChI |
InChI=1S/C257H383N65O77S6/c1-29-131(23)205(313-193(339)104-259)252(393)317-204(130(21)22)248(389)288-159(75-82-200(349)350)217(358)282-156(71-78-189(263)335)221(362)308-183-116-403-404-117-184-243(384)305-178(111-324)240(381)294-162(88-123(7)8)225(366)295-168(95-140-53-61-146(329)62-54-140)228(369)283-154(69-76-187(261)333)218(359)290-161(87-122(5)6)223(364)285-158(74-81-199(347)348)220(361)302-174(101-190(264)336)235(376)298-170(97-142-57-65-148(331)66-58-142)231(372)309-182(242(383)304-176(255(396)397)103-192(266)338)115-402-401-114-181(214(355)273-107-194(340)278-153(72-79-197(343)344)216(357)281-151(51-42-84-271-257(267)268)212(353)272-108-195(341)279-166(93-138-46-36-32-37-47-138)227(368)297-167(94-139-48-38-33-39-49-139)230(371)299-171(98-143-59-67-149(332)68-60-143)238(379)320-208(135(27)327)254(395)322-85-43-52-186(322)246(387)286-152(50-40-41-83-258)222(363)321-209(136(28)328)256(398)399)311-250(391)203(129(19)20)316-236(377)164(90-125(11)12)292-229(370)169(96-141-55-63-147(330)64-56-141)296-224(365)160(86-121(3)4)289-210(351)133(25)277-215(356)157(73-80-198(345)346)287-247(388)202(128(17)18)315-237(378)165(91-126(13)14)293-233(374)173(100-145-106-270-120-276-145)301-239(380)177(110-323)280-196(342)109-274-213(354)180(113-400-405-118-185(310-244(183)385)245(386)319-207(134(26)326)253(394)306-179(112-325)241(382)318-206(132(24)30-2)251(392)312-184)307-226(367)163(89-124(9)10)291-232(373)172(99-144-105-269-119-275-144)300-219(360)155(70-77-188(262)334)284-234(375)175(102-191(265)337)303-249(390)201(127(15)16)314-211(352)150(260)92-137-44-34-31-35-45-137/h31-39,44-49,53-68,105-106,119-136,150-186,201-209,323-332H,29-30,40-43,50-52,69-104,107-118,258-260H2,1-28H3,(H2,261,333)(H2,262,334)(H2,263,335)(H2,264,336)(H2,265,337)(H2,266,338)(H,269,275)(H,270,276)(H,272,353)(H,273,355)(H,274,354)(H,277,356)(H,278,340)(H,279,341)(H,280,342)(H,281,357)(H,282,358)(H,283,369)(H,284,375)(H,285,364)(H,286,387)(H,287,388)(H,288,389)(H,289,351)(H,290,359)(H,291,373)(H,292,370)(H,293,374)(H,294,381)(H,295,366)(H,296,365)(H,297,368)(H,298,376)(H,299,371)(H,300,360)(H,301,380)(H,302,361)(H,303,390)(H,304,383)(H,305,384)(H,306,394)(H,307,367)(H,308,362)(H,309,372)(H,310,385)(H,311,391)(H,312,392)(H,313,339)(H,314,352)(H,315,378)(H,316,377)(H,317,393)(H,318,382)(H,319,386)(H,320,379)(H,321,363)(H,343,344)(H,345,346)(H,347,348)(H,349,350)(H,396,397)(H,398,399)(H4,267,268,271)/t131-,132-,133-,134+,135+,136+,150-,151-,152-,153-,154-,155-,156-,157-,158-,159-,160-,161-,162-,163-,164-,165-,166-,167-,168-,169-,170-,171-,172-,173-,174-,175-,176-,177-,178-,179-,180-,181-,182-,183-,184-,185-,186-,201-,202-,203-,204-,205-,206-,207-,208-,209-/m0/s1 |
Clé InChI |
PBGKTOXHQIOBKM-FHFVDXKLSA-N |
SMILES isomérique |
CC[C@H](C)[C@H]1C(=O)N[C@H]2CSSC[C@@H](C(=O)N[C@@H](CSSC[C@@H](C(=O)NCC(=O)N[C@H](C(=O)N[C@H](C(=O)N[C@H](C(=O)N[C@H](C(=O)N[C@H](C(=O)N[C@H](C(=O)N[C@H](C(=O)N[C@H](C(=O)N[C@H](C(=O)N[C@H](C(=O)N[C@@H](CSSC[C@H](NC(=O)[C@@H](NC(=O)[C@@H](NC(=O)[C@@H](NC(=O)[C@@H](NC(=O)[C@@H](NC(=O)[C@@H](NC(=O)[C@@H](NC(=O)[C@@H](NC2=O)CO)CC(C)C)CC3=CC=C(C=C3)O)CCC(=O)N)CC(C)C)CCC(=O)O)CC(=O)N)CC4=CC=C(C=C4)O)C(=O)N[C@@H](CC(=O)N)C(=O)O)C(=O)NCC(=O)N[C@@H](CCC(=O)O)C(=O)N[C@@H](CCCNC(=N)N)C(=O)NCC(=O)N[C@@H](CC5=CC=CC=C5)C(=O)N[C@@H](CC6=CC=CC=C6)C(=O)N[C@@H](CC7=CC=C(C=C7)O)C(=O)N[C@@H]([C@@H](C)O)C(=O)N8CCC[C@H]8C(=O)N[C@@H](CCCCN)C(=O)N[C@@H]([C@@H](C)O)C(=O)O)C(C)C)CC(C)C)CC9=CC=C(C=C9)O)CC(C)C)C)CCC(=O)O)C(C)C)CC(C)C)CC2=CNC=N2)CO)NC(=O)[C@H](CC(C)C)NC(=O)[C@H](CC2=CNC=N2)NC(=O)[C@H](CCC(=O)N)NC(=O)[C@H](CC(=O)N)NC(=O)[C@H](C(C)C)NC(=O)[C@H](CC2=CC=CC=C2)N)C(=O)N[C@H](C(=O)N[C@H](C(=O)N1)CO)[C@@H](C)O)NC(=O)[C@H](CCC(=O)N)NC(=O)[C@H](CCC(=O)O)NC(=O)[C@H](C(C)C)NC(=O)[C@H]([C@@H](C)CC)NC(=O)CN |
SMILES |
CCC(C)C1C(=O)NC2CSSCC(C(=O)NC(CSSCC(C(=O)NCC(=O)NC(C(=O)NC(C(=O)NC(C(=O)NC(C(=O)NC(C(=O)NC(C(=O)NC(C(=O)NC(C(=O)NC(C(=O)NC(C(=O)NC(CSSCC(NC(=O)C(NC(=O)C(NC(=O)C(NC(=O)C(NC(=O)C(NC(=O)C(NC(=O)C(NC(=O)C(NC2=O)CO)CC(C)C)CC3=CC=C(C=C3)O)CCC(=O)N)CC(C)C)CCC(=O)O)CC(=O)N)CC4=CC=C(C=C4)O)C(=O)NC(CC(=O)N)C(=O)O)C(=O)NCC(=O)NC(CCC(=O)O)C(=O)NC(CCCNC(=N)N)C(=O)NCC(=O)NC(CC5=CC=CC=C5)C(=O)NC(CC6=CC=CC=C6)C(=O)NC(CC7=CC=C(C=C7)O)C(=O)NC(C(C)O)C(=O)N8CCCC8C(=O)NC(CCCCN)C(=O)NC(C(C)O)C(=O)O)C(C)C)CC(C)C)CC9=CC=C(C=C9)O)CC(C)C)C)CCC(=O)O)C(C)C)CC(C)C)CC2=CNC=N2)CO)NC(=O)C(CC(C)C)NC(=O)C(CC2=CNC=N2)NC(=O)C(CCC(=O)N)NC(=O)C(CC(=O)N)NC(=O)C(C(C)C)NC(=O)C(CC2=CC=CC=C2)N)C(=O)NC(C(=O)NC(C(=O)N1)CO)C(C)O)NC(=O)C(CCC(=O)N)NC(=O)C(CCC(=O)O)NC(=O)C(C(C)C)NC(=O)C(C(C)CC)NC(=O)CN |
SMILES canonique |
CCC(C)C1C(=O)NC2CSSCC(C(=O)NC(CSSCC(C(=O)NCC(=O)NC(C(=O)NC(C(=O)NC(C(=O)NC(C(=O)NC(C(=O)NC(C(=O)NC(C(=O)NC(C(=O)NC(C(=O)NC(C(=O)NC(CSSCC(NC(=O)C(NC(=O)C(NC(=O)C(NC(=O)C(NC(=O)C(NC(=O)C(NC(=O)C(NC(=O)C(NC2=O)CO)CC(C)C)CC3=CC=C(C=C3)O)CCC(=O)N)CC(C)C)CCC(=O)O)CC(=O)N)CC4=CC=C(C=C4)O)C(=O)NC(CC(=O)N)C(=O)O)C(=O)NCC(=O)NC(CCC(=O)O)C(=O)NC(CCCNC(=N)N)C(=O)NCC(=O)NC(CC5=CC=CC=C5)C(=O)NC(CC6=CC=CC=C6)C(=O)NC(CC7=CC=C(C=C7)O)C(=O)NC(C(C)O)C(=O)N8CCCC8C(=O)NC(CCCCN)C(=O)NC(C(C)O)C(=O)O)C(C)C)CC(C)C)CC9=CC=C(C=C9)O)CC(C)C)C)CCC(=O)O)C(C)C)CC(C)C)CC2=CNC=N2)CO)NC(=O)C(CC(C)C)NC(=O)C(CC2=CNC=N2)NC(=O)C(CCC(=O)N)NC(=O)C(CC(=O)N)NC(=O)C(C(C)C)NC(=O)C(CC2=CC=CC=C2)N)C(=O)NC(C(=O)NC(C(=O)N1)CO)C(C)O)NC(=O)C(CCC(=O)N)NC(=O)C(CCC(=O)O)NC(=O)C(C(C)C)NC(=O)C(C(C)CC)NC(=O)CN |
Origine du produit |
United States |
Avertissement et informations sur les produits de recherche in vitro
Veuillez noter que tous les articles et informations sur les produits présentés sur BenchChem sont destinés uniquement à des fins informatives. Les produits disponibles à l'achat sur BenchChem sont spécifiquement conçus pour des études in vitro, qui sont réalisées en dehors des organismes vivants. Les études in vitro, dérivées du terme latin "in verre", impliquent des expériences réalisées dans des environnements de laboratoire contrôlés à l'aide de cellules ou de tissus. Il est important de noter que ces produits ne sont pas classés comme médicaments et n'ont pas reçu l'approbation de la FDA pour la prévention, le traitement ou la guérison de toute condition médicale, affection ou maladie. Nous devons souligner que toute forme d'introduction corporelle de ces produits chez les humains ou les animaux est strictement interdite par la loi. Il est essentiel de respecter ces directives pour assurer la conformité aux normes légales et éthiques en matière de recherche et d'expérimentation.