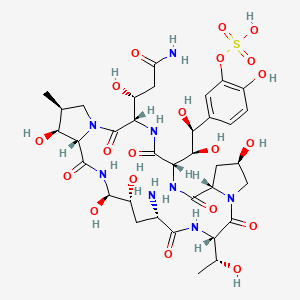
FR179642
Vue d'ensemble
Description
FR179642 est un composé intermédiaire clé utilisé dans la synthèse du médicament antifongique Micafungin, qui appartient à la classe des échinocandines . Il sert de noyau peptidique cyclique pour le lipopeptide antifongique FR901379, structurellement similaire . Le composé est connu pour son rôle dans la lutte contre les infections fongiques, ce qui en fait une molécule importante dans le domaine de la chimie médicinale.
Applications De Recherche Scientifique
FR179642 a plusieurs applications en recherche scientifique :
Mécanisme d'Action
This compound exerce ses effets en servant de précurseur au Micafungin, qui inhibe la 1,3-β-glucane synthase, une enzyme nécessaire à la synthèse des parois cellulaires fongiques . Cette inhibition perturbe la formation de la paroi cellulaire, ce qui entraîne la mort des cellules fongiques . Les cibles moléculaires et les voies impliquées comprennent le noyau peptidique cyclique et la structure lipopeptidique antifongique de type échinocandine .
Mécanisme D'action
Target of Action
FR179642, also known as Pneumocandin M1 or FR-179642, is a key intermediate in the synthesis of the echinocandin antifungal Micafungin . Echinocandins are a class of antifungal drugs that inhibit the synthesis of 1,3-β-D-glucan in the fungal cell wall . Therefore, the primary target of this compound is the 1,3-β-D-glucan synthase, an enzyme critical for fungal cell wall synthesis.
Mode of Action
This compound, as an echinocandin, interacts with its target, the 1,3-β-D-glucan synthase, by inhibiting its activity. This inhibition disrupts the synthesis of 1,3-β-D-glucan, a crucial component of the fungal cell wall. The disruption in the cell wall synthesis leads to osmotic instability and ultimately, the death of the fungal cell .
Biochemical Pathways
The primary biochemical pathway affected by this compound is the 1,3-β-D-glucan synthesis pathway in fungi. By inhibiting the 1,3-β-D-glucan synthase, this compound prevents the formation of 1,3-β-D-glucan, leading to a weakened fungal cell wall and increased susceptibility to osmotic pressure . This results in the lysis of the fungal cell, thereby exerting its antifungal effect.
Pharmacokinetics
Micafungin is known for its good water solubility, which contributes to its bioavailability
Analyse Biochimique
Biochemical Properties
This enzyme deacylates the acyl side chain of FR901379 to yield FR179642 . The interaction between this compound and this enzyme is crucial for the synthesis of Micafungin .
Cellular Effects
As an intermediate in the synthesis of Micafungin, it plays a crucial role in the production of this antifungal agent .
Molecular Mechanism
The molecular mechanism of this compound involves its interaction with cyclic lipopeptide acylase . This enzyme deacylates the acyl side chain of FR901379, yielding this compound . This process is a key step in the synthesis of Micafungin .
Temporal Effects in Laboratory Settings
It is known that this compound is a stable compound used as a key intermediate in the synthesis of Micafungin .
Metabolic Pathways
This compound is involved in the metabolic pathway leading to the synthesis of Micafungin . It is produced through the deacylation of the acyl side chain of FR901379 by cyclic lipopeptide acylase .
Subcellular Localization
As an intermediate in the synthesis of Micafungin, it is likely to be found in the locations where this synthesis occurs .
Méthodes De Préparation
La préparation de FR179642 implique plusieurs étapes, axées principalement sur la désacylation de FR901379. Voici une voie de synthèse détaillée :
Fermentation : Le processus commence par la fermentation de la bactérie Actinoplanes utahensis pour obtenir la désacylase FR901379.
Désacylation : FR901379 est dissous dans du méthanol à une concentration de 3,0 à 5,0 g/L et mélangé à la désacylase.
Séparation et purification : Après 12 à 48 heures de réaction, this compound est séparé et purifié.
Cette méthode est efficace, rentable et permet d'obtenir du this compound de haute pureté .
Analyse Des Réactions Chimiques
FR179642 subit diverses réactions chimiques, notamment :
Oxydation : Le composé peut être oxydé dans des conditions spécifiques pour former différents produits oxydés.
Réduction : Des réactions de réduction peuvent être effectuées à l'aide d'agents réducteurs courants pour produire des formes réduites du composé.
Substitution : Les réactions de substitution impliquent le remplacement d'un groupe fonctionnel par un autre, souvent à l'aide de réactifs tels que les halogènes ou les groupes alkyles.
Les principaux produits formés à partir de ces réactions dépendent des réactifs et des conditions spécifiques utilisés .
Comparaison Avec Des Composés Similaires
FR179642 est unique par rapport aux autres composés similaires en raison de son rôle spécifique d'intermédiaire dans la synthèse du Micafungin. Les composés similaires comprennent :
FR901379 : Le composé parent dont est dérivé this compound.
Micafungin : Le produit antifongique final synthétisé à l'aide de this compound.
Autres échinocandines : Composés tels que la caspofungine et l'anidulafungine, qui appartiennent également à la classe des échinocandines mais ont des structures et des voies de synthèse différentes.
Ces composés partagent des propriétés antifongiques similaires mais diffèrent par leurs structures chimiques et leurs applications spécifiques .
Propriétés
IUPAC Name |
[5-[(1S,2S)-2-[(3S,6S,9S,11R,15S,18S,20R,21R,24S,25S,26S)-18-amino-3-[(1R)-3-amino-1-hydroxy-3-oxopropyl]-11,20,21,25-tetrahydroxy-15-[(1R)-1-hydroxyethyl]-26-methyl-2,5,8,14,17,23-hexaoxo-1,4,7,13,16,22-hexazatricyclo[22.3.0.09,13]heptacosan-6-yl]-1,2-dihydroxyethyl]-2-hydroxyphenyl] hydrogen sulfate | |
---|---|---|
Source | PubChem | |
URL | https://pubchem.ncbi.nlm.nih.gov | |
Description | Data deposited in or computed by PubChem | |
InChI |
InChI=1S/C35H52N8O20S/c1-11-9-43-25(26(11)50)33(57)41-31(55)19(48)7-15(36)29(53)38-22(12(2)44)34(58)42-10-14(45)6-16(42)30(54)40-24(32(56)39-23(35(43)59)18(47)8-21(37)49)28(52)27(51)13-3-4-17(46)20(5-13)63-64(60,61)62/h3-5,11-12,14-16,18-19,22-28,31,44-48,50-52,55H,6-10,36H2,1-2H3,(H2,37,49)(H,38,53)(H,39,56)(H,40,54)(H,41,57)(H,60,61,62)/t11-,12+,14+,15-,16-,18+,19+,22-,23-,24-,25-,26-,27-,28-,31+/m0/s1 | |
Source | PubChem | |
URL | https://pubchem.ncbi.nlm.nih.gov | |
Description | Data deposited in or computed by PubChem | |
InChI Key |
QCYMOOBOFFUBHZ-CPYYHODSSA-N | |
Source | PubChem | |
URL | https://pubchem.ncbi.nlm.nih.gov | |
Description | Data deposited in or computed by PubChem | |
Canonical SMILES |
CC1CN2C(C1O)C(=O)NC(C(CC(C(=O)NC(C(=O)N3CC(CC3C(=O)NC(C(=O)NC(C2=O)C(CC(=O)N)O)C(C(C4=CC(=C(C=C4)O)OS(=O)(=O)O)O)O)O)C(C)O)N)O)O | |
Source | PubChem | |
URL | https://pubchem.ncbi.nlm.nih.gov | |
Description | Data deposited in or computed by PubChem | |
Isomeric SMILES |
C[C@H]1CN2[C@@H]([C@H]1O)C(=O)N[C@@H]([C@@H](C[C@@H](C(=O)N[C@H](C(=O)N3C[C@@H](C[C@H]3C(=O)N[C@H](C(=O)N[C@H](C2=O)[C@@H](CC(=O)N)O)[C@@H]([C@H](C4=CC(=C(C=C4)O)OS(=O)(=O)O)O)O)O)[C@@H](C)O)N)O)O | |
Source | PubChem | |
URL | https://pubchem.ncbi.nlm.nih.gov | |
Description | Data deposited in or computed by PubChem | |
Molecular Formula |
C35H52N8O20S | |
Source | PubChem | |
URL | https://pubchem.ncbi.nlm.nih.gov | |
Description | Data deposited in or computed by PubChem | |
Molecular Weight |
936.9 g/mol | |
Source | PubChem | |
URL | https://pubchem.ncbi.nlm.nih.gov | |
Description | Data deposited in or computed by PubChem | |
CAS No. |
168110-44-9 | |
Record name | FR-179642 | |
Source | ChemIDplus | |
URL | https://pubchem.ncbi.nlm.nih.gov/substance/?source=chemidplus&sourceid=0168110449 | |
Description | ChemIDplus is a free, web search system that provides access to the structure and nomenclature authority files used for the identification of chemical substances cited in National Library of Medicine (NLM) databases, including the TOXNET system. | |
Record name | FR-179642 | |
Source | FDA Global Substance Registration System (GSRS) | |
URL | https://gsrs.ncats.nih.gov/ginas/app/beta/substances/44677O2TDO | |
Description | The FDA Global Substance Registration System (GSRS) enables the efficient and accurate exchange of information on what substances are in regulated products. Instead of relying on names, which vary across regulatory domains, countries, and regions, the GSRS knowledge base makes it possible for substances to be defined by standardized, scientific descriptions. | |
Explanation | Unless otherwise noted, the contents of the FDA website (www.fda.gov), both text and graphics, are not copyrighted. They are in the public domain and may be republished, reprinted and otherwise used freely by anyone without the need to obtain permission from FDA. Credit to the U.S. Food and Drug Administration as the source is appreciated but not required. | |
Q1: What is the significance of using macroporous resin in the extraction of FR179642?
A1: The research paper highlights the use of macroporous resin for the adsorption and desorption of this compound from the fermentation broth of Coleophoma sp. []. This method offers a significant advantage as it simplifies the extraction process and makes it suitable for industrial-scale production. Macroporous resins are known for their high binding capacity and selectivity, contributing to a more efficient purification process.
Q2: How does reverse-phase packing material contribute to obtaining high-purity this compound?
A2: Following the initial extraction using macroporous resin, the crude extract of this compound undergoes further purification using reverse-phase packing material for chromatography []. This technique separates compounds based on their different affinities for the stationary phase (packing material) and the mobile phase (solvent). The specific interaction of this compound with the chosen reverse-phase packing material enables its effective separation from other compounds in the crude extract, resulting in a high-purity product.
Avertissement et informations sur les produits de recherche in vitro
Veuillez noter que tous les articles et informations sur les produits présentés sur BenchChem sont destinés uniquement à des fins informatives. Les produits disponibles à l'achat sur BenchChem sont spécifiquement conçus pour des études in vitro, qui sont réalisées en dehors des organismes vivants. Les études in vitro, dérivées du terme latin "in verre", impliquent des expériences réalisées dans des environnements de laboratoire contrôlés à l'aide de cellules ou de tissus. Il est important de noter que ces produits ne sont pas classés comme médicaments et n'ont pas reçu l'approbation de la FDA pour la prévention, le traitement ou la guérison de toute condition médicale, affection ou maladie. Nous devons souligner que toute forme d'introduction corporelle de ces produits chez les humains ou les animaux est strictement interdite par la loi. Il est essentiel de respecter ces directives pour assurer la conformité aux normes légales et éthiques en matière de recherche et d'expérimentation.