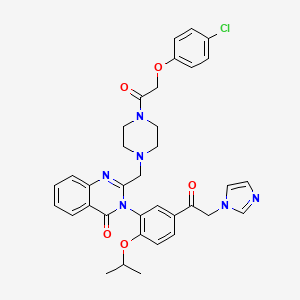
Imidazole ketone erastin
- Cliquez sur DEMANDE RAPIDE pour recevoir un devis de notre équipe d'experts.
- Avec des produits de qualité à un prix COMPÉTITIF, vous pouvez vous concentrer davantage sur votre recherche.
Vue d'ensemble
Description
Imidazole Ketone Erastin (IKE) is an inducer of ferroptosis . It inhibits glutamate release in human CCF-STTG1 astrocytoma cells , indicating inhibition of the system xc- cystine/glutamate transporter . It is a potent, selective, and metabolically stable inhibitor of the cystine-glutamate antiporter, system xc-, and an activator of ferroptosis . IKE has anti-tumor activity .
Synthesis Analysis
IKE is identified as a potent and metabolically stable inhibitor of system xc− and inducer of ferroptosis . The authors show that IKE exerts an anti-tumor effect in a mouse xenograft model and exhibits distinct lipid metabolism effects . The review highlights the recent advances in the regiocontrolled synthesis of substituted imidazoles .
Molecular Structure Analysis
The structure of erastin-bound xCT–4F2hc complex reveals molecular mechanisms underlying erastin-induced ferroptosis . Erastin inhibits system Xc− -mediated cystine transport and induces ferroptosis .
Chemical Reactions Analysis
IKE is a potent, selective, and metabolically stable system xc− inhibitor . IKE inhibited tumor growth in a diffuse large B cell lymphoma mouse model . The small molecule IKE is an erastin analog incorporating a carbonyl that can potentially form a reversible covalent interaction with proteins, resulting in >1003 potency improvement compared with erastin in some cell lines .
Physical And Chemical Properties Analysis
IKE has a molecular weight of 655.14 and is soluble in DMSO at 90 mg/mL . It is stored at -20°C for 3 years in powder form and at 4°C for 2 years in solvent .
Applications De Recherche Scientifique
1. Induction of Ferroptosis in Photodynamic Therapy IKE is used as a ferroptosis inducer in photodynamic therapy (PDT). PDT is an effective tumor treatment that involves the administration of a photosensitizer to generate cytotoxic reactive oxygen species (ROS) from molecular oxygen that is produced from energy absorption following tumor irradiation at specific wavelengths . IKE enhances the antitumor effect of PDT by inducing ferroptosis via accumulation of lipid peroxidation .
Enhancement of Antitumor Effect
IKE has been shown to enhance the antitumor effect of talaporfin sodium-photodynamic therapy (TS-PDT). This is achieved by inducing ferroptosis, a form of regulated cell death, through the disruption of the glutathione peroxidase 4 (GPX4) antioxidant system, leading to lipid peroxidation .
Inhibition of System xc−
IKE is a potent and metabolically stable inhibitor of system xc−, a cystine-glutamate transporter. By inhibiting system xc−, IKE prevents cystine uptake, thereby causing ferroptosis by depleting reduced glutathione (GSH) .
4. Slowing Tumor Growth in Mouse Lymphoma Model IKE has been found to slow tumor growth in a mouse lymphoma model. It exerts an antitumor effect by inhibiting system xc−, leading to glutathione depletion, lipid peroxidation, and the induction of ferroptosis biomarkers .
Potential Anticancer Drug
IKE and its analogs have been explored as anticancer drugs by triggering ferroptosis in cancer cells .
Accelerating Wound Healing
Topical administration of IKE, along with early-stage apoptotic cells, to mice after full-thickness skin wounding markedly accelerated wound healing and decreased the apoptotic cell burden in the wound .
Mécanisme D'action
Target of Action
Imidazole Ketone Erastin (IKE) primarily targets the cystine-glutamate antiporter, system xc- . This system plays a crucial role in maintaining the balance of cystine and glutamate in cells . IKE acts as a potent and metabolically stable inhibitor of system xc-, disrupting this balance .
Mode of Action
IKE interacts with its target, system xc-, by inhibiting its function . This inhibition leads to a decrease in the uptake of cystine, an essential amino acid for the synthesis of glutathione . The reduction in glutathione levels results in an increase in lipid peroxidation, a key feature of a regulated cell death process known as ferroptosis .
Biochemical Pathways
IKE’s action affects the glutathione and lipid metabolism pathways . By inhibiting system xc-, IKE causes a depletion of glutathione, a critical antioxidant in cells . This depletion leads to an increase in lipid peroxidation, a process where lipids in the cell membrane undergo oxidative degradation . The increase in lipid peroxidation is a characteristic feature of ferroptosis .
Pharmacokinetics
IKE is described as a metabolically stable compound, making it potentially suitable for in vivo applications . .
Result of Action
The primary result of IKE’s action is the induction of ferroptosis, a form of regulated cell death . In a mouse lymphoma model, IKE was shown to slow tumor growth by inducing ferroptosis . This was evidenced by the presence of ferroptosis biomarkers, glutathione depletion, and increased lipid peroxidation .
Action Environment
The action, efficacy, and stability of IKE can be influenced by various environmental factors. For instance, the pH of the environment can affect IKE’s solubility . .
Safety and Hazards
IKE may form combustible dust concentrations in air. It is harmful if swallowed and causes severe skin burns and eye damage. It may cause respiratory irritation and may damage the unborn child . It is advised to obtain special instructions before use and not to handle until all safety precautions have been read and understood .
Orientations Futures
IKE and piperazine erastin (PE) have poor solubility in water and relative lability, so the solubility should be taken into consideration when drugs are designed . Erastin analogs are capable of inhibiting tumor growth, and they have minimal toxicity both in vitro and in vivo . While small molecule system xc− inhibitors are promising agents for inducing ferroptosis in cancers that are highly dependent on cystine import, there are a number of potential concerns in translating such agents for therapeutic benefit .
Propriétés
IUPAC Name |
2-[[4-[2-(4-chlorophenoxy)acetyl]piperazin-1-yl]methyl]-3-[5-(2-imidazol-1-ylacetyl)-2-propan-2-yloxyphenyl]quinazolin-4-one |
Source
|
---|---|---|
Source | PubChem | |
URL | https://pubchem.ncbi.nlm.nih.gov | |
Description | Data deposited in or computed by PubChem | |
InChI |
InChI=1S/C35H35ClN6O5/c1-24(2)47-32-12-7-25(31(43)20-40-14-13-37-23-40)19-30(32)42-33(38-29-6-4-3-5-28(29)35(42)45)21-39-15-17-41(18-16-39)34(44)22-46-27-10-8-26(36)9-11-27/h3-14,19,23-24H,15-18,20-22H2,1-2H3 |
Source
|
Source | PubChem | |
URL | https://pubchem.ncbi.nlm.nih.gov | |
Description | Data deposited in or computed by PubChem | |
InChI Key |
PSPXJPWGVFNGQI-UHFFFAOYSA-N |
Source
|
Source | PubChem | |
URL | https://pubchem.ncbi.nlm.nih.gov | |
Description | Data deposited in or computed by PubChem | |
Canonical SMILES |
CC(C)OC1=C(C=C(C=C1)C(=O)CN2C=CN=C2)N3C(=NC4=CC=CC=C4C3=O)CN5CCN(CC5)C(=O)COC6=CC=C(C=C6)Cl |
Source
|
Source | PubChem | |
URL | https://pubchem.ncbi.nlm.nih.gov | |
Description | Data deposited in or computed by PubChem | |
Molecular Formula |
C35H35ClN6O5 |
Source
|
Source | PubChem | |
URL | https://pubchem.ncbi.nlm.nih.gov | |
Description | Data deposited in or computed by PubChem | |
Molecular Weight |
655.1 g/mol |
Source
|
Source | PubChem | |
URL | https://pubchem.ncbi.nlm.nih.gov | |
Description | Data deposited in or computed by PubChem | |
Product Name |
Imidazole ketone erastin |
Q & A
Q1: What is the primary mechanism of action of Imidazole Ketone Erastin?
A1: Imidazole Ketone Erastin (IKE) functions as a potent inhibitor of the cystine/glutamate antiporter system xc-, also known as system xc-. [, , , , ] This system plays a critical role in maintaining cellular redox balance by importing cystine for glutathione synthesis. By inhibiting system xc-, IKE disrupts glutathione production, leading to the accumulation of reactive oxygen species (ROS) and ultimately triggering ferroptosis. [, , , , , , ]
Q2: What are the downstream effects of IKE-mediated system xc- inhibition?
A2: IKE's inhibition of system xc- triggers a cascade of events culminating in ferroptosis:
- Depletion of intracellular Glutathione: System xc- inhibition prevents cystine uptake, leading to a decline in glutathione (GSH) levels. [, , , , , ]
- Reduced Glutathione Peroxidase 4 (GPX4) Activity: GSH depletion impairs the function of GPX4, an enzyme crucial for detoxifying lipid peroxides. [, , , , ]
- Lipid Peroxidation and Ferroptosis: Uncontrolled lipid peroxidation ensues due to impaired GPX4 activity, leading to membrane damage and ultimately ferroptotic cell death. [, , , , , ]
Q3: What makes IKE a potent inducer of ferroptosis compared to other system xc- inhibitors?
A3: While the exact reasons are still under investigation, IKE exhibits superior potency compared to its parent compound, Erastin. This enhanced activity is likely attributed to structural modifications, specifically the imidazole ketone moiety, which may influence its binding affinity to system xc- or its cellular uptake and distribution. [, ]
Q4: What is the molecular formula and weight of Imidazole Ketone Erastin?
A4: Unfortunately, the provided abstracts do not contain the precise molecular formula and weight of Imidazole Ketone Erastin. For detailed structural information, it is recommended to refer to the complete research articles or chemical databases.
Q5: Does Imidazole Ketone Erastin interact with drug transporters, and how might this impact its efficacy?
A5: Research indicates that IKE is a substrate for P-glycoprotein (P-gp), a multidrug resistance transporter. [, ] Overexpression of P-gp in cancer cells can confer resistance to IKE, potentially hindering its therapeutic efficacy. [, ] This highlights the importance of considering drug-transporter interactions when developing IKE-based therapies.
Q6: What evidence supports the efficacy of Imidazole Ketone Erastin in preclinical models of cancer?
A6: IKE demonstrates promising antitumor activity in various preclinical models. For instance, IKE effectively inhibits tumor growth in a mouse lymphoma model. [] In a subcutaneous xenograft model of Diffuse Large B Cell Lymphoma (DLBCL), IKE encapsulated in biodegradable nanoparticles exhibits enhanced delivery and therapeutic efficacy. [] These findings underscore IKE's potential as a novel anticancer agent.
Q7: Are there known resistance mechanisms to Imidazole Ketone Erastin, and how do they relate to other ferroptosis inducers or anticancer agents?
A7: One established resistance mechanism involves the overexpression of P-glycoprotein (P-gp), which can efflux IKE from cells, reducing its intracellular concentration and effectiveness. [, ] This resistance mechanism is not unique to IKE and is commonly observed with various anticancer drugs. Further research is crucial to elucidate other potential resistance mechanisms and explore strategies to circumvent them.
Avertissement et informations sur les produits de recherche in vitro
Veuillez noter que tous les articles et informations sur les produits présentés sur BenchChem sont destinés uniquement à des fins informatives. Les produits disponibles à l'achat sur BenchChem sont spécifiquement conçus pour des études in vitro, qui sont réalisées en dehors des organismes vivants. Les études in vitro, dérivées du terme latin "in verre", impliquent des expériences réalisées dans des environnements de laboratoire contrôlés à l'aide de cellules ou de tissus. Il est important de noter que ces produits ne sont pas classés comme médicaments et n'ont pas reçu l'approbation de la FDA pour la prévention, le traitement ou la guérison de toute condition médicale, affection ou maladie. Nous devons souligner que toute forme d'introduction corporelle de ces produits chez les humains ou les animaux est strictement interdite par la loi. Il est essentiel de respecter ces directives pour assurer la conformité aux normes légales et éthiques en matière de recherche et d'expérimentation.