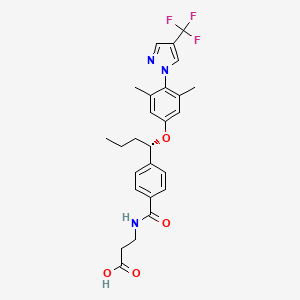
Antagonistes du récepteur du glucagon-4
Vue d'ensemble
Description
Les antagonistes du récepteur du glucagon-4 sont une classe de composés conçus pour inhiber l'action du glucagon, une hormone qui élève la glycémie en favorisant la néoglucogenèse et la glycogénolyse dans le foie. Ces antagonistes sont principalement étudiés pour leur potentiel dans le traitement du diabète de type 2 en réduisant l'hyperglycémie et en améliorant le contrôle glycémique .
Applications De Recherche Scientifique
Glucagon receptor antagonists-4 have a wide range of scientific research applications:
Chemistry: Used as model compounds to study receptor-ligand interactions and develop new synthetic methodologies.
Biology: Investigated for their role in modulating metabolic pathways and hormone signaling.
Medicine: Explored as potential therapeutic agents for treating type 2 diabetes and other metabolic disorders by reducing hyperglycemia and improving insulin sensitivity
Méthodes De Préparation
Voies de synthèse et conditions de réaction : La synthèse des antagonistes du récepteur du glucagon-4 implique généralement une synthèse organique à plusieurs étapes. Une approche courante comprend la formation d'une structure de base, suivie de modifications de groupe fonctionnel pour améliorer l'affinité de liaison au récepteur et la sélectivité.
Méthodes de production industrielle : La production industrielle des this compound implique une synthèse organique à grande échelle utilisant des réacteurs automatisés et des mesures strictes de contrôle de la qualité. Le processus comprend souvent des étapes de purification telles que la cristallisation et la chromatographie pour garantir la pureté et l'efficacité du produit final .
Analyse Des Réactions Chimiques
Types de réactions : Les antagonistes du récepteur du glucagon-4 subissent diverses réactions chimiques, notamment :
Oxydation : Introduction d'atomes d'oxygène pour former des oxydes.
Réduction : Élimination d'atomes d'oxygène ou addition d'atomes d'hydrogène.
Substitution : Remplacement d'un groupe fonctionnel par un autre.
Réactifs et conditions courants : Les réactifs courants utilisés dans ces réactions comprennent des agents oxydants comme le permanganate de potassium, des agents réducteurs comme le borohydrure de sodium et des réactifs de substitution comme les halogénoalcanes. Les conditions de réaction impliquent souvent des températures et des niveaux de pH contrôlés pour optimiser le rendement et la sélectivité .
Principaux produits formés : Les principaux produits formés à partir de ces réactions sont généralement des dérivés de la structure de base avec des propriétés pharmacologiques améliorées, telles qu'une affinité de liaison au récepteur accrue et une stabilité métabolique améliorée .
4. Applications de la recherche scientifique
Les this compound ont un large éventail d'applications de recherche scientifique :
Chimie : Utilisés comme composés modèles pour étudier les interactions récepteur-ligand et développer de nouvelles méthodologies de synthèse.
Biologie : Étudiés pour leur rôle dans la modulation des voies métaboliques et de la signalisation hormonale.
Médecine : Explorés comme agents thérapeutiques potentiels pour le traitement du diabète de type 2 et d'autres troubles métaboliques en réduisant l'hyperglycémie et en améliorant la sensibilité à l'insuline
5. Mécanisme d'action
Le mécanisme d'action des this compound implique la liaison au récepteur du glucagon, bloquant ainsi l'interaction du récepteur avec le glucagon. Cette inhibition empêche l'activation des voies de signalisation en aval qui favorisent la néoglucogenèse et la glycogénolyse dans le foie. En bloquant ces voies, les this compound réduisent la production hépatique de glucose et abaissent la glycémie .
Composés similaires :
Antagonistes du récepteur du glucagon-1, 2 et 3 : Ces composés partagent un mécanisme d'action similaire, mais peuvent différer en termes d'affinité de liaison, de sélectivité et de propriétés pharmacocinétiques.
Agonistes du récepteur du peptide-1 de type glucagon : Ces composés ciblent également la signalisation du glucagon, mais agissent en augmentant la sécrétion d'insuline et en réduisant la libération de glucagon.
Unicité : Les this compound sont uniques dans leur capacité à inhiber sélectivement le récepteur du glucagon sans affecter les autres récepteurs apparentés. Cette sélectivité réduit le risque d'effets hors cible et améliore le potentiel thérapeutique du composé .
Mécanisme D'action
The mechanism of action of glucagon receptor antagonists-4 involves binding to the glucagon receptor, thereby blocking the receptor’s interaction with glucagon. This inhibition prevents the activation of downstream signaling pathways that promote gluconeogenesis and glycogenolysis in the liver. By blocking these pathways, glucagon receptor antagonists-4 reduce hepatic glucose production and lower blood glucose levels .
Comparaison Avec Des Composés Similaires
Glucagon receptor antagonists-1, 2, and 3: These compounds share a similar mechanism of action but may differ in their binding affinity, selectivity, and pharmacokinetic properties.
Glucagon-like peptide-1 receptor agonists: These compounds also target glucagon signaling but act by enhancing insulin secretion and reducing glucagon release.
Uniqueness: Glucagon receptor antagonists-4 are unique in their ability to selectively inhibit the glucagon receptor without affecting other related receptors. This selectivity reduces the risk of off-target effects and improves the compound’s therapeutic potential .
Propriétés
IUPAC Name |
3-[[4-[(1S)-1-[3,5-dimethyl-4-[4-(trifluoromethyl)pyrazol-1-yl]phenoxy]butyl]benzoyl]amino]propanoic acid | |
---|---|---|
Source | PubChem | |
URL | https://pubchem.ncbi.nlm.nih.gov | |
Description | Data deposited in or computed by PubChem | |
InChI |
InChI=1S/C26H28F3N3O4/c1-4-5-22(18-6-8-19(9-7-18)25(35)30-11-10-23(33)34)36-21-12-16(2)24(17(3)13-21)32-15-20(14-31-32)26(27,28)29/h6-9,12-15,22H,4-5,10-11H2,1-3H3,(H,30,35)(H,33,34)/t22-/m0/s1 | |
Source | PubChem | |
URL | https://pubchem.ncbi.nlm.nih.gov | |
Description | Data deposited in or computed by PubChem | |
InChI Key |
IBDYYOQKQCCSDP-QFIPXVFZSA-N | |
Source | PubChem | |
URL | https://pubchem.ncbi.nlm.nih.gov | |
Description | Data deposited in or computed by PubChem | |
Canonical SMILES |
CCCC(C1=CC=C(C=C1)C(=O)NCCC(=O)O)OC2=CC(=C(C(=C2)C)N3C=C(C=N3)C(F)(F)F)C | |
Source | PubChem | |
URL | https://pubchem.ncbi.nlm.nih.gov | |
Description | Data deposited in or computed by PubChem | |
Isomeric SMILES |
CCC[C@@H](C1=CC=C(C=C1)C(=O)NCCC(=O)O)OC2=CC(=C(C(=C2)C)N3C=C(C=N3)C(F)(F)F)C | |
Source | PubChem | |
URL | https://pubchem.ncbi.nlm.nih.gov | |
Description | Data deposited in or computed by PubChem | |
Molecular Formula |
C26H28F3N3O4 | |
Source | PubChem | |
URL | https://pubchem.ncbi.nlm.nih.gov | |
Description | Data deposited in or computed by PubChem | |
Molecular Weight |
503.5 g/mol | |
Source | PubChem | |
URL | https://pubchem.ncbi.nlm.nih.gov | |
Description | Data deposited in or computed by PubChem | |
CAS No. |
1393124-08-7 | |
Record name | PF-06291874 | |
Source | ChemIDplus | |
URL | https://pubchem.ncbi.nlm.nih.gov/substance/?source=chemidplus&sourceid=1393124087 | |
Description | ChemIDplus is a free, web search system that provides access to the structure and nomenclature authority files used for the identification of chemical substances cited in National Library of Medicine (NLM) databases, including the TOXNET system. | |
Record name | PF-06291874 | |
Source | DrugBank | |
URL | https://www.drugbank.ca/drugs/DB15065 | |
Description | The DrugBank database is a unique bioinformatics and cheminformatics resource that combines detailed drug (i.e. chemical, pharmacological and pharmaceutical) data with comprehensive drug target (i.e. sequence, structure, and pathway) information. | |
Explanation | Creative Common's Attribution-NonCommercial 4.0 International License (http://creativecommons.org/licenses/by-nc/4.0/legalcode) | |
Record name | PF-06291874 | |
Source | FDA Global Substance Registration System (GSRS) | |
URL | https://gsrs.ncats.nih.gov/ginas/app/beta/substances/CGY4I8F278 | |
Description | The FDA Global Substance Registration System (GSRS) enables the efficient and accurate exchange of information on what substances are in regulated products. Instead of relying on names, which vary across regulatory domains, countries, and regions, the GSRS knowledge base makes it possible for substances to be defined by standardized, scientific descriptions. | |
Explanation | Unless otherwise noted, the contents of the FDA website (www.fda.gov), both text and graphics, are not copyrighted. They are in the public domain and may be republished, reprinted and otherwise used freely by anyone without the need to obtain permission from FDA. Credit to the U.S. Food and Drug Administration as the source is appreciated but not required. | |
Synthesis routes and methods I
Procedure details
Synthesis routes and methods II
Procedure details
Retrosynthesis Analysis
AI-Powered Synthesis Planning: Our tool employs the Template_relevance Pistachio, Template_relevance Bkms_metabolic, Template_relevance Pistachio_ringbreaker, Template_relevance Reaxys, Template_relevance Reaxys_biocatalysis model, leveraging a vast database of chemical reactions to predict feasible synthetic routes.
One-Step Synthesis Focus: Specifically designed for one-step synthesis, it provides concise and direct routes for your target compounds, streamlining the synthesis process.
Accurate Predictions: Utilizing the extensive PISTACHIO, BKMS_METABOLIC, PISTACHIO_RINGBREAKER, REAXYS, REAXYS_BIOCATALYSIS database, our tool offers high-accuracy predictions, reflecting the latest in chemical research and data.
Strategy Settings
Precursor scoring | Relevance Heuristic |
---|---|
Min. plausibility | 0.01 |
Model | Template_relevance |
Template Set | Pistachio/Bkms_metabolic/Pistachio_ringbreaker/Reaxys/Reaxys_biocatalysis |
Top-N result to add to graph | 6 |
Feasible Synthetic Routes
Avertissement et informations sur les produits de recherche in vitro
Veuillez noter que tous les articles et informations sur les produits présentés sur BenchChem sont destinés uniquement à des fins informatives. Les produits disponibles à l'achat sur BenchChem sont spécifiquement conçus pour des études in vitro, qui sont réalisées en dehors des organismes vivants. Les études in vitro, dérivées du terme latin "in verre", impliquent des expériences réalisées dans des environnements de laboratoire contrôlés à l'aide de cellules ou de tissus. Il est important de noter que ces produits ne sont pas classés comme médicaments et n'ont pas reçu l'approbation de la FDA pour la prévention, le traitement ou la guérison de toute condition médicale, affection ou maladie. Nous devons souligner que toute forme d'introduction corporelle de ces produits chez les humains ou les animaux est strictement interdite par la loi. Il est essentiel de respecter ces directives pour assurer la conformité aux normes légales et éthiques en matière de recherche et d'expérimentation.