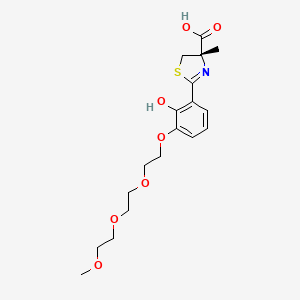
Deferitazole
Vue d'ensemble
Description
Le déférritazole, également connu sous les noms de FBS-0701 et SPD-602, est un dérivé de polyéther qui est entré en phase 1 et 2 des essais cliniques. Il est conçu pour chélater le fer, en ciblant spécifiquement les ions fer (III). Le composé présente une forte affinité et une sélectivité pour le fer (III), ce qui en fait un chélateur de fer thérapeutique potentiel .
Analyse Des Réactions Chimiques
Le déférritazole participe à diverses réactions chimiques, notamment la liaison avec les métaux trivalents et la récupération du fer provenant de sources labiles. Voici quelques points clés :
Affinité pour le fer (III) : Le déférritazole présente une valeur log β2 de 33,39 ± 0,03, ce qui indique une forte liaison aux ions fer (III).
Autres métaux trivalents : Il se lie également à Al (III) et La (III) avec une forte affinité.
Cations divalents : Son affinité pour les cations divalents est légèrement inférieure, sauf pour Cu (II), qui a une valeur log β2 de 25,5.
Récupération du fer : Le déférritazole récupère efficacement le fer du citrate et de l'albumine, comme les autres chélateurs de fer thérapeutiques.
4. Applications de recherche scientifique
Le déférritazole a été étudié dans divers contextes :
Bêta-thalassémie : Étudié pour son potentiel dans le traitement de la surcharge en fer transfusionnelle.
Insuffisance hépatique et insuffisance rénale : Exploré pour ses effets dans ces conditions.
Propriétés antipaludiques : Bien qu'il ne soit pas directement mentionné, ses propriétés chélatrices du fer peuvent avoir des implications dans la recherche sur le paludisme.
5. Mécanisme d'action
Le mécanisme exact par lequel le déférritazole exerce ses effets reste à éclaircir. Il implique probablement la séquestration du fer en excès, empêchant ses effets nocifs et le stress oxydatif. Les cibles moléculaires et les voies impliquées nécessitent des recherches supplémentaires.
Applications De Recherche Scientifique
Deferitazole has been studied in various contexts:
Beta-thalassemia: Investigated for its potential in treating transfusional iron overload.
Hepatic Impairment and Impaired Renal Function: Explored for its effects in these conditions.
Antimalarial Properties: Although not directly mentioned, its iron-chelating properties may have implications in malaria research.
Mécanisme D'action
The exact mechanism by which deferitazole exerts its effects remains to be fully elucidated. it likely involves sequestering excess iron, preventing its harmful effects and oxidative stress. Molecular targets and pathways involved need further investigation.
Comparaison Avec Des Composés Similaires
La singularité du déférritazole réside dans sa forte affinité pour le fer (III) et sa stabilité dans des conditions physiologiques. Parmi les composés similaires, on peut citer le déférasirox, la défériprone et la desferrioxamine, mais les propriétés distinctes du déférritazole en font un candidat prometteur pour la thérapie de chélation du fer.
Méthodes De Préparation
Les voies de synthèse et les conditions de réaction du déférritazole n'ont pas été largement rapportées. Il convient de noter que le déférritazole a fait l'objet de recherches systématiques et d'optimisation basées sur les analogues de la desferrithiocine. Des détails supplémentaires sur ses méthodes de production industrielle ne sont pas facilement disponibles.
Propriétés
Numéro CAS |
945635-15-4 |
---|---|
Formule moléculaire |
C18H25NO7S |
Poids moléculaire |
399.5 g/mol |
Nom IUPAC |
(4S)-2-[2-hydroxy-3-[2-[2-(2-methoxyethoxy)ethoxy]ethoxy]phenyl]-4-methyl-5H-1,3-thiazole-4-carboxylic acid |
InChI |
InChI=1S/C18H25NO7S/c1-18(17(21)22)12-27-16(19-18)13-4-3-5-14(15(13)20)26-11-10-25-9-8-24-7-6-23-2/h3-5,20H,6-12H2,1-2H3,(H,21,22)/t18-/m1/s1 |
Clé InChI |
AWHIMFSHNAAMBM-GOSISDBHSA-N |
SMILES isomérique |
C[C@@]1(CSC(=N1)C2=C(C(=CC=C2)OCCOCCOCCOC)O)C(=O)O |
SMILES |
O=C([C@]1(C)N=C(C2=CC=CC(OCCOCCOCCOC)=C2O)SC1)O |
SMILES canonique |
CC1(CSC(=N1)C2=C(C(=CC=C2)OCCOCCOCCOC)O)C(=O)O |
Apparence |
Solid powder |
Pureté |
>98% (or refer to the Certificate of Analysis) |
Durée de conservation |
>2 years if stored properly |
Solubilité |
Soluble in DMSO, not in water |
Stockage |
Dry, dark and at 0 - 4 C for short term (days to weeks) or -20 C for long term (months to years). |
Synonymes |
FBS-0701; FBS0701; FBS 0701; SPD-602; SPD 602; SPD602; Deferitazole |
Origine du produit |
United States |
Synthesis routes and methods
Procedure details
Retrosynthesis Analysis
AI-Powered Synthesis Planning: Our tool employs the Template_relevance Pistachio, Template_relevance Bkms_metabolic, Template_relevance Pistachio_ringbreaker, Template_relevance Reaxys, Template_relevance Reaxys_biocatalysis model, leveraging a vast database of chemical reactions to predict feasible synthetic routes.
One-Step Synthesis Focus: Specifically designed for one-step synthesis, it provides concise and direct routes for your target compounds, streamlining the synthesis process.
Accurate Predictions: Utilizing the extensive PISTACHIO, BKMS_METABOLIC, PISTACHIO_RINGBREAKER, REAXYS, REAXYS_BIOCATALYSIS database, our tool offers high-accuracy predictions, reflecting the latest in chemical research and data.
Strategy Settings
Precursor scoring | Relevance Heuristic |
---|---|
Min. plausibility | 0.01 |
Model | Template_relevance |
Template Set | Pistachio/Bkms_metabolic/Pistachio_ringbreaker/Reaxys/Reaxys_biocatalysis |
Top-N result to add to graph | 6 |
Feasible Synthetic Routes
Avertissement et informations sur les produits de recherche in vitro
Veuillez noter que tous les articles et informations sur les produits présentés sur BenchChem sont destinés uniquement à des fins informatives. Les produits disponibles à l'achat sur BenchChem sont spécifiquement conçus pour des études in vitro, qui sont réalisées en dehors des organismes vivants. Les études in vitro, dérivées du terme latin "in verre", impliquent des expériences réalisées dans des environnements de laboratoire contrôlés à l'aide de cellules ou de tissus. Il est important de noter que ces produits ne sont pas classés comme médicaments et n'ont pas reçu l'approbation de la FDA pour la prévention, le traitement ou la guérison de toute condition médicale, affection ou maladie. Nous devons souligner que toute forme d'introduction corporelle de ces produits chez les humains ou les animaux est strictement interdite par la loi. Il est essentiel de respecter ces directives pour assurer la conformité aux normes légales et éthiques en matière de recherche et d'expérimentation.