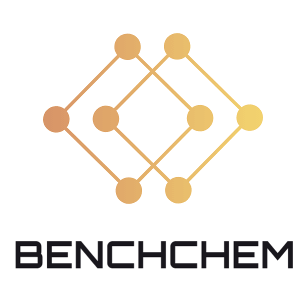
Fucoidan
Vue d'ensemble
Description
Fucoidan is a long chain sulfated polysaccharide found in various species of brown algae . It is commonly extracted from seaweed species like Fucus vesiculosus, Cladosiphon okamuranus, Laminaria japonica, and Undaria pinnatifida . This compound has been utilized in a range of therapeutic health care preparations, being incorporated as high-value ingredients in nutritional, medical device, skincare, and dermatological products .
Synthesis Analysis
This compound’s synthesis involves the extraction from more than a hundred different species of seaweed . The fractions with low charge correspond mostly to highly heterogeneous fucoidans, containing other monosaccharides like xylose, galactose, mannose, rhamnose, and glucuronic acid . This compound derivatives, whose basic sugar chains are composed of repeating α (1,4)-linked L-fucopyranosyl residues with different sulfation patterns, were designed and systematically synthesized .Molecular Structure Analysis
This compound is characterized by two structural types: Type I this compound has repeating units of α- (1→3)-linked α-L-fucopyranose and Type II this compound has alternately repeating units of α- (1→3)- and α- (1→4)-linked α-L-fucopyranose .Chemical Reactions Analysis
This compound’s chemical reactions are influenced by its extraction and purification conditions . Enzymes catalyzing partial cleavage of seaweed polysaccharides, for instance, fucoidanase and sulfatase, have been proposed to be useful tools for investigating the structural features and biological activity–function relationship of this compound polysaccharides .Physical and Chemical Properties Analysis
This compound’s physical and chemical properties are influenced by its extraction and purification conditions . It is characterized by possessing sulfate ester groups that impart negatively charged surfaces, low/high molecular weight, and water solubility .Applications De Recherche Scientifique
Liver Disease Applications
Fucoidan, a sulfur-rich polysaccharide from brown algae, shows significant potential in liver disease treatment. It has been studied for its role in various liver conditions, including viral hepatitis, liver fibrosis, liver cancer, nonalcoholic fatty liver, and liver injury induced by drugs and ischemia. The mechanisms underlying its protective effects involve modulation of oxidative stress, carbohydrate and lipid metabolism, inflammatory responses, tumor proliferation, and metastasis, making it a promising candidate for liver protection and drug development (Li, Guo, & Wu, 2020).
Cardiovascular System Applications
This compound exhibits various biological activities that are beneficial for cardiovascular diseases, including anticoagulant, hypolipidemic, anti-thrombotic, anti-inflammatory, immunomodulatory, anti-tumor, anti-adhesive, and anti-hypertensive properties. Studies have mainly focused on its anti-inflammatory effects, but its pro-angiogenic and pro-vasculogenic properties also offer promising therapeutic potential for cardiovascular diseases (Zaporozhets & Besednova, 2016).
Anticancer Applications
This compound's anticancer properties have been a significant focus of research. It has shown antiproliferative, antiangiogenic, and anticancer properties in vitro, targeting multiple receptors or signaling molecules in tumor cells and immune cells. Its low toxicity makes it a suitable agent for cancer prevention or treatment. However, further in vivo examination in animal tumor models is required for its development as a marine anticancer drug (Kwak, 2014).
Nanomedicine Applications
In the field of nanomedicine, this compound has been used for developing therapeutic and diagnostic nanosystems. Its applications include use as imaging agents, drug carriers, and exploiting its intrinsic properties. The need for reproducible, well-characterized this compound fractions is essential for significant progress in this area (Chollet et al., 2016).
Metabolic Disease Applications
This compound has demonstrated therapeutic potential in metabolic diseases, such as hypertension, diabetes, obesity, and dyslipidemia. Clinical trials and preclinical research have reported its antihypertensive, antidiabetic, antiobesity, and hypolipidemic effects, offering a promising avenue for alternative therapies in metabolic disorders (Méndez-Del Villar et al., 2022).
Mécanisme D'action
Target of Action:
Fucoidan is a polysaccharide derived from brown algae and marine invertebrates. It primarily consists of sulfated fucose residues. While it has a wide range of biological activities, one of its key targets is cancer cells .
Action Environment:
Environmental factors (such as pH, temperature, and salinity) influence this compound’s stability and efficacy. Additionally, its interactions with other compounds affect its overall action.
Orientations Futures
Fucoidan’s future developments will be based on well-informed choices due to a wide range of information that is gradually accumulating . Two clinical trials are underway, focused on the biodistribution and tolerance of this compound . The aspects that need to be considered regarding this compound-based treatments and future directions for the treatment of BC have been indicated .
Analyse Biochimique
Biochemical Properties
Fucoidan interacts with various enzymes, proteins, and other biomolecules. It has been reported that this compound can directly exert anti-cancer actions through cell cycle arrest, induction of apoptosis, and can also indirectly kill cancer cells by activating natural killer cells, macrophages, etc . The biochemical properties of this compound are linked to its degree of sulfation, monosaccharide composition, and molecular weight .
Cellular Effects
This compound has shown to have significant effects on various types of cells and cellular processes. It influences cell function by impacting cell signaling pathways, gene expression, and cellular metabolism . For instance, it has been reported that this compound can inhibit the growth of breast cancer cells, liver cancer cells, and cervical cancer cells by inducing apoptosis (cell death) and cell cycle arrest .
Molecular Mechanism
This compound exerts its effects at the molecular level through various mechanisms. It binds to pattern-recognition receptors (PRRs), a group of membrane receptors including scavenger receptors (SRs), Toll-like receptors (TLRs), complement receptor 3 (CR3, aMb2-integrin, CD11b/CD18), C-type lectin receptors (CLRs), mannose receptor (MR) or other target molecules to trigger intracellular signaling cascades, mediating cellular physiological mechanisms .
Temporal Effects in Laboratory Settings
The effects of this compound change over time in laboratory settings. It has been reported that pretreatment with this compound can reduce neutrophil infiltration by 70–90% at early time points . This suggests that this compound has a significant impact on cellular function over time.
Dosage Effects in Animal Models
The effects of this compound vary with different dosages in animal models. For instance, a study showed that daily this compound supplementation (Undaria pinnatifida extract containing >85% this compound, 1 g/day) for three weeks led to significant pain reduction . Another study reported that this compound at a dosage of 50 mg/kg body weight targeted kidney and liver, reaching concentrations of 1092.31 and 284.27 μg/g respectively after 0.5 h .
Metabolic Pathways
This compound is involved in various metabolic pathways. It has been reported that this compound modulates hyperlipidemia by inhibiting cholesterol and aliphatic acid synthesis, accelerating the mitochondrial β-oxidation or peroxisomal oxidation degradation of aliphatic acid in vivo .
Transport and Distribution
This compound is transported and distributed within cells and tissues. A study showed that this compound is transported via clathrin-mediated endocytosis . After injection of 50 mg/kg body weight into the tail vein of mice, this compound targeted kidney and liver .
Subcellular Localization
It is known that this compound binds to certain receptors such as pattern-recognition receptors (PRRs), which may influence protein structure, function, and localization .
Propriétés
{ "Design of the Synthesis Pathway": "The synthesis pathway of Fucoidan involves the extraction of fucoidan from brown seaweed followed by purification and modification of the extracted compound.", "Starting Materials": [ "Brown seaweed (source of fucoidan)", "Water", "Ethanol", "Acetone", "Hydrochloric acid", "Sodium hydroxide", "Enzymes (e.g. cellulase, pectinase)" ], "Reaction": [ "Extraction of fucoidan from brown seaweed using water or a mixture of water and ethanol", "Purification of fucoidan using acetone precipitation and dialysis", "Modification of fucoidan using hydrochloric acid or sodium hydroxide to adjust the pH and enzymatic treatment to remove impurities", "Further modification of fucoidan using chemical or enzymatic methods to introduce specific functional groups or to increase its bioactivity" ] } | |
Numéro CAS |
9072-19-9 |
Formule moléculaire |
(C6H9O3SO3)n |
Poids moléculaire |
256.27 |
InChI |
InChI=1S/C8H16O7S/c1-4-7(13-3)6(9)8(5(2)14-4)15-16(10,11)12/h4-9H,1-3H3,(H,10,11,12)/t4-,5+,6+,7-,8-/m1/s1 |
Clé InChI |
WZPKXSSMRAQGAC-DWOUCZDBSA-N |
SMILES |
C[C@]1([H])[C@@H](OC)[C@H](O)[C@H](OS(O)(=O)=O)[C@H](C)O1 |
Synonymes |
Fucan; Sulfated α-L-Fucan |
Origine du produit |
United States |
Retrosynthesis Analysis
AI-Powered Synthesis Planning: Our tool employs the Template_relevance Pistachio, Template_relevance Bkms_metabolic, Template_relevance Pistachio_ringbreaker, Template_relevance Reaxys, Template_relevance Reaxys_biocatalysis model, leveraging a vast database of chemical reactions to predict feasible synthetic routes.
One-Step Synthesis Focus: Specifically designed for one-step synthesis, it provides concise and direct routes for your target compounds, streamlining the synthesis process.
Accurate Predictions: Utilizing the extensive PISTACHIO, BKMS_METABOLIC, PISTACHIO_RINGBREAKER, REAXYS, REAXYS_BIOCATALYSIS database, our tool offers high-accuracy predictions, reflecting the latest in chemical research and data.
Strategy Settings
Precursor scoring | Relevance Heuristic |
---|---|
Min. plausibility | 0.01 |
Model | Template_relevance |
Template Set | Pistachio/Bkms_metabolic/Pistachio_ringbreaker/Reaxys/Reaxys_biocatalysis |
Top-N result to add to graph | 6 |
Feasible Synthetic Routes
A: Fucoidan is a term used to describe a diverse family of sulfated polysaccharides found in the cell walls of various species of brown seaweed [, , ]. These polysaccharides are primarily composed of L-fucose and sulfate ester groups, but their exact structure and composition can vary widely depending on the species of seaweed, its geographic location, and the extraction method used [, , ].
A: The biological activities of this compound are closely tied to its structural features, including molecular weight, sulfate content, monosaccharide composition, and glycosidic linkages [, , , ]. For example, research has shown that lower molecular weight this compound fractions often exhibit stronger anti-cancer activity than higher molecular weight fractions []. Additionally, the degree of sulfation has been linked to the antioxidant and anti-inflammatory properties of this compound, with highly sulfated fractions demonstrating greater potency [, , ].
ANone: this compound has been shown to interact with a variety of molecular targets, resulting in a diverse range of downstream effects.
A: Due to the heterogeneous nature of this compound, providing a single molecular formula or weight is not possible. The molecular weight of this compound varies widely depending on the source and extraction method, ranging from a few kDa to over 2000 kDa [, , ].
ANone: Yes, several spectroscopic techniques are commonly used to characterize this compound, including Fourier Transform Infrared Spectroscopy (FTIR) and Nuclear Magnetic Resonance (NMR) spectroscopy.
A: The stability of this compound can vary depending on factors such as temperature, pH, and the presence of enzymes or other degrading agents. Generally, this compound is relatively stable under acidic conditions but can be degraded under alkaline conditions [, ]. Additionally, high temperatures can lead to the desulfation and depolymerization of this compound, potentially affecting its biological activity.
ANone: Yes, several formulation approaches have been investigated to enhance the delivery and efficacy of this compound.
ANone: A combination of analytical techniques is typically employed to characterize and quantify this compound:
ANone: The increasing demand for this compound has raised concerns about the sustainability of its extraction from wild seaweed populations.
A: While this compound possesses a unique combination of structural features and biological activities, other marine-derived polysaccharides, such as carrageenans, alginates, and ulvans, share some overlapping properties and could be explored as potential alternatives or substitutes depending on the specific application [].
Avertissement et informations sur les produits de recherche in vitro
Veuillez noter que tous les articles et informations sur les produits présentés sur BenchChem sont destinés uniquement à des fins informatives. Les produits disponibles à l'achat sur BenchChem sont spécifiquement conçus pour des études in vitro, qui sont réalisées en dehors des organismes vivants. Les études in vitro, dérivées du terme latin "in verre", impliquent des expériences réalisées dans des environnements de laboratoire contrôlés à l'aide de cellules ou de tissus. Il est important de noter que ces produits ne sont pas classés comme médicaments et n'ont pas reçu l'approbation de la FDA pour la prévention, le traitement ou la guérison de toute condition médicale, affection ou maladie. Nous devons souligner que toute forme d'introduction corporelle de ces produits chez les humains ou les animaux est strictement interdite par la loi. Il est essentiel de respecter ces directives pour assurer la conformité aux normes légales et éthiques en matière de recherche et d'expérimentation.