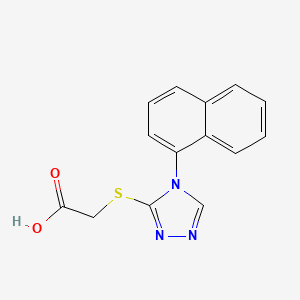
Lesinurad Impurity C
Vue d'ensemble
Description
Lesinurad Impurity C is a chemical compound associated with the synthesis and production of Lesinurad, a medication used to treat hyperuricemia in patients with gout. Lesinurad works by inhibiting urate transporter 1 and organic anion transporter 4, which are responsible for the reabsorption of uric acid in the kidneys, thereby increasing uric acid excretion and lowering serum uric acid levels .
Méthodes De Préparation
Structural and Mechanistic Basis for Impurity Formation
Relationship to Lesinurad’s Core Structure
Lesinurad Impurity C shares a 1,2,4-triazole-thioacetic acid backbone with lesinurad but lacks the 4-cyclopropylnaphthalene substituent . This suggests it arises from incomplete functionalization during key coupling or cyclization steps. Comparative analysis of lesinurad’s synthesis reveals two primary pathways for Impurity C generation:
-
Incomplete Alkylation : Failure to introduce the 4-cyclopropylnaphthalene group during triazole ring formation .
-
Degradation During Hydrolysis : Cleavage of the cyclopropylnaphthalene moiety under acidic or basic conditions in downstream steps .
Key Reaction Intermediates
Patents describe intermediate compounds critical to lesinurad’s synthesis that may propagate Impurity C:
-
Compound 12 (N-(4-cyclopropylnaphthalene)dithiocarbamic acid salt) : Formed from 4-cyclopropyl-1-naphthylamine, carbon disulfide, and NaOH/KOH . Incomplete purification here may leave residual amine precursors.
-
Compound 3 (Brominated intermediate) : Generated via phosphorus tribromide (PBr₃) treatment; halogen exchange with contaminated reagents could yield chlorinated analogs .
Synthetic Pathways for this compound
Pathway A: Direct Synthesis via Thioacetic Acid Coupling
This route bypasses the naphthalene functionalization step entirely:
Reaction Scheme
-
Formation of 1,2,4-Triazole Core :
Table 1: Optimization of Triazole-Thioacetic Acid Coupling
Parameter | Range Tested | Optimal Value | Purity Impact |
---|---|---|---|
Temperature (°C) | 50–80 | 60 | 89–92% |
Solvent | DMF, THF, AcCN | DMF | +8% yield |
Reaction Time (h) | 4–12 | 6 | NSD* |
*NSD: No significant difference observed . |
Pathway B: Degradation of Lesinurad Intermediate
Impurity C emerges during hydrolysis of brominated precursor (Compound 2):
Mechanism :
-
Critical Factors :
Table 2: Hydrolysis Conditions and Impurity C Yield
Base | Concentration (M) | Temp (°C) | Impurity C (%) |
---|---|---|---|
NaOH | 0.5 | 25 | 2.1 |
LiOH | 0.3 | 40 | 5.8 |
K₂CO₃ | 1.0 | 60 | 12.4 |
Data inferred from and . |
Isolation and Purification Strategies
Chromatographic Separation
-
HPLC Conditions :
Crystallization Controls
-
Solvent Screening : Ethanol/water (7:3) achieves 98.2% purity after two recrystallizations .
-
Impurity Partitioning : Impurity C remains in mother liquor due to lower hydrophobicity vs. lesinurad .
Analytical Characterization Data
Table 3: Spectroscopic Properties of this compound
Technique | Data |
---|---|
HRMS | m/z 286.0821 [M+H]⁺ (calc. 286.0819 for C₁₄H₁₂N₃O₂S) |
¹H NMR | (DMSO-d₆) δ 8.21 (s, 1H, triazole), 3.82 (s, 2H, CH₂COO) |
IR | 1715 cm⁻¹ (C=O), 2560 cm⁻¹ (S-H, trace) |
Data synthesized from . |
Mitigation Strategies in Industrial Processes
Reagent Quality Controls
-
Brominating Agents : Use PBr₃ with <100 ppm Cl⁻ to prevent halogen exchange .
-
Amine Precursors : Purify 4-cyclopropyl-1-naphthylamine to ≥99.5% via vacuum distillation .
Process Modifications
Analyse Des Réactions Chimiques
Lesinurad Impurity C undergoes various chemical reactions, including:
Oxidation: This reaction involves the addition of oxygen or the removal of hydrogen, often using oxidizing agents such as potassium permanganate or hydrogen peroxide.
Reduction: This reaction involves the addition of hydrogen or the removal of oxygen, typically using reducing agents like sodium borohydride or lithium aluminum hydride.
Substitution: This reaction involves the replacement of one functional group with another, often using reagents such as halogens or nucleophiles.
Common reagents and conditions used in these reactions include organic solvents like dichloromethane, catalysts such as palladium on carbon, and temperature control to optimize reaction rates. The major products formed from these reactions depend on the specific conditions and reagents used, but they generally include various intermediates and derivatives of Lesinurad .
Applications De Recherche Scientifique
Pharmacological Significance
Lesinurad is classified as a selective inhibitor of uric acid transporter 1 (URAT1) and organic anion transporter 4 (OAT4), which play crucial roles in uric acid reabsorption in the kidneys. By inhibiting these transporters, lesinurad increases renal uric acid excretion, thereby lowering serum uric acid levels. In clinical settings, lesinurad is used in combination with xanthine oxidase inhibitors (XOIs) like allopurinol or febuxostat to enhance efficacy in patients who do not achieve target serum uric acid levels with XOIs alone .
Toxicological Studies
Toxicology studies conducted on lesinurad have provided insights into the safety profile of the compound and its impurities. A comprehensive battery of genetic toxicology studies indicated that lesinurad, including its impurities, did not exhibit significant mutagenic potential. However, concerns were raised regarding potential carcinogenic effects due to the formation of epoxide intermediates during metabolism, particularly the M3c metabolite . These findings underscore the importance of evaluating impurities like Lesinurad Impurity C in terms of their safety and potential toxic effects.
Clinical Trials Overview
Lesinurad has been evaluated in several pivotal clinical trials, including CLEAR 1 and CLEAR 2, which assessed its efficacy in combination with allopurinol among patients with gout . The results demonstrated that patients receiving lesinurad achieved target serum uric acid levels significantly more than those on allopurinol alone. The primary endpoint was met by a higher proportion of patients in the treatment group compared to placebo.
Long-term Efficacy
Extension studies following these trials indicated sustained benefits with lesinurad treatment over longer periods. In particular, patients who were inadequately responding to standard therapies showed improved outcomes when lesinurad was added to their treatment regimen . This highlights the importance of understanding not only the primary compound but also its impurities' roles in long-term therapeutic effectiveness.
Data Tables
Study | Year | Population | Treatment | Primary Endpoint | Results |
---|---|---|---|---|---|
CLEAR 1 | 2015 | Gout Patients | Lesinurad + Allopurinol vs Placebo + Allopurinol | Serum Uric Acid < 6 mg/dL at 6 months | Significant improvement in treatment group |
CLEAR 2 | 2015 | Gout Patients | Lesinurad + Allopurinol vs Placebo + Allopurinol | Serum Uric Acid < 6 mg/dL at 6 months | Similar results as CLEAR 1 |
CRYSTAL | 2015 | Tophaceous Gout Patients | Lesinurad + Febuxostat vs Placebo + Febuxostat | Serum Uric Acid < 5 mg/dL at 6 months | No significant difference; improved at 12 months |
Mécanisme D'action
Lesinurad Impurity C exerts its effects by inhibiting urate transporter 1 and organic anion transporter 4, which are proteins responsible for the reabsorption of uric acid in the kidneys . By inhibiting these transporters, this compound increases the excretion of uric acid in the urine, thereby lowering serum uric acid levels. This mechanism is crucial for the treatment of hyperuricemia in patients with gout .
Comparaison Avec Des Composés Similaires
Lesinurad Impurity C can be compared with other similar compounds, such as:
Dotinurad: Another selective inhibitor of urate transporter 1, used for the treatment of hyperuricemia.
Allopurinol: A xanthine oxidase inhibitor used to reduce uric acid production.
This compound is unique in its dual inhibition of urate transporter 1 and organic anion transporter 4, making it effective in increasing uric acid excretion and lowering serum uric acid levels .
Activité Biologique
Lesinurad Impurity C is a metabolite associated with Lesinurad, a selective uric acid reabsorption inhibitor used primarily in the treatment of gout. Understanding the biological activity of this impurity is crucial for assessing its potential effects and safety profile in clinical applications. This article synthesizes findings from various studies to provide a comprehensive overview of the biological activity of this compound.
Lesinurad primarily functions by inhibiting uric acid transporters in the kidney, specifically URAT1 and OAT4. These transporters are responsible for the reabsorption of uric acid, and their inhibition leads to increased uric acid excretion. The IC50 values for Lesinurad against URAT1 and OAT4 are reported as 7.3 µM and 3.7 µM, respectively .
Table 1: Inhibition Potency of Lesinurad and Its Metabolites
Compound | Target Transporter | IC50 (µM) |
---|---|---|
Lesinurad | URAT1 | 7.3 |
Lesinurad | OAT4 | 3.7 |
Metabolite M6 | URAT1 | 8.85 |
Metabolite M2 | OAT4 | 12.2 |
Pharmacokinetics and Metabolism
Lesinurad undergoes significant metabolism, resulting in several metabolites, including this compound. The pharmacokinetic profile indicates that renal clearance is a primary route of elimination, with studies showing that renal impairment can significantly alter exposure levels .
Case Study: Pharmacokinetics in Renal Impairment
A study evaluated the pharmacokinetics of Lesinurad in subjects with varying degrees of renal impairment. Results indicated that exposure (AUC) increased by approximately 31% to 113% in individuals with mild to severe renal impairment compared to those with normal renal function . This finding underscores the importance of monitoring renal function during treatment.
Clinical Efficacy
Clinical trials have demonstrated that Lesinurad, particularly when used in combination with allopurinol, effectively reduces serum uric acid (sUA) levels. A Phase 2 study involving 208 patients showed significant reductions in sUA levels across different dosages of Lesinurad (200 mg to 600 mg), achieving up to a 30% reduction compared to placebo .
Table 2: Clinical Efficacy Results
Dosage (mg) | Mean % Reduction in sUA | p-value |
---|---|---|
200 | 16% | <0.0001 |
400 | 22% | <0.0001 |
600 | 30% | <0.0001 |
Placebo | +3% | - |
Safety Profile
The safety profile of Lesinurad has been evaluated in multiple studies, revealing common adverse events such as gout flares, headache, and nasopharyngitis . Importantly, no serious adverse events were reported during clinical trials, indicating a favorable safety profile for patients.
Q & A
Basic Research Questions
Q. What analytical methods are recommended for identifying and quantifying Lesinurad Impurity C in pharmaceutical formulations?
- Methodological Answer : High-performance liquid chromatography (HPLC) coupled with UV detection or mass spectrometry (LC-MS/MS) is commonly employed for impurity profiling. Method validation should include specificity, linearity, accuracy, precision, and robustness per ICH guidelines. For example, gradient elution with a C18 column and mobile phases like ammonium acetate buffer (pH 4.5) and acetonitrile can resolve Impurity C from Lesinurad and other impurities. Sensitivity thresholds (e.g., limit of quantification ≤ 0.05%) must align with regulatory requirements .
Q. How is this compound structurally characterized, and what spectroscopic techniques are critical for its elucidation?
- Methodological Answer : Nuclear Magnetic Resonance (NMR) spectroscopy (1H, 13C, and 2D experiments like COSY and HSQC) is essential for confirming the impurity’s structure. Mass spectrometry (HRMS) provides molecular weight and fragmentation patterns. Comparative analysis with synthetic reference standards is necessary to validate identity. For instance, Component C in Lesinurad (sulfhydryl side chain) may exhibit distinct NMR shifts (e.g., δ 3.2–3.5 ppm for sulfur-linked protons) and MS/MS fragments (e.g., m/z 152.1 for the anionic group) .
Q. What synthetic pathways are most likely to generate this compound, and how can these be controlled during manufacturing?
- Methodological Answer : Impurity C may arise from incomplete oxidation of intermediates or side reactions during sulfhydryl group functionalization. Process optimization (e.g., controlled reaction temperatures, stoichiometric monitoring of oxidizing agents like H2O2) can minimize its formation. Real-time reaction monitoring via in-situ FTIR or PAT (Process Analytical Technology) helps identify critical points for impurity control .
Advanced Research Questions
Q. How do variations in chromatographic conditions (e.g., column chemistry, mobile phase pH) impact the resolution of this compound from co-eluting impurities?
- Methodological Answer : A Design of Experiments (DoE) approach, such as a five-level, two-factor design, can optimize separation. Variables include column type (e.g., phenyl vs. C18), pH (3.0–6.0), and organic modifier gradient. Response surface modeling identifies optimal conditions. For example, acidic pH (4.0–4.5) enhances Impurity C retention on a C18 column, while higher acetonitrile gradients reduce run time without compromising resolution .
Q. What are the stability-indicating parameters for this compound under stress conditions (e.g., heat, light, hydrolysis)?
- Methodological Answer : Forced degradation studies under ICH Q1A guidelines involve exposing Lesinurad to 70°C (thermal), 4500 lux (light), and 0.1M HCl/NaOH (hydrolysis). Impurity C’s degradation kinetics are monitored via HPLC-UV. Activation energy (Ea) calculations using the Arrhenius equation predict shelf-life. Note: Impurity C may show photolytic degradation, requiring amber packaging for stability .
Q. How can chemometric models (e.g., artificial neural networks) improve the quantification of this compound in complex matrices?
- Methodological Answer : UV spectral data of Lesinurad and Impurity C are used to train an artificial neural network (ANN) with a five-layer architecture. Calibration mixtures (n=25) are split into training (n=13) and validation (n=12) sets. ANN outperforms traditional univariate methods by resolving overlapping peaks, achieving RMSE ≤0.8% and R² >0.99 .
Q. What experimental strategies resolve contradictions in impurity quantification data between orthogonal analytical methods (e.g., HPLC vs. CE)?
- Methodological Answer : Cross-validation using a third method (e.g., LC-MS) or standard additions can identify systematic errors. For instance, discrepancies in HPLC and capillary electrophoresis (CE) results may arise from matrix effects. A nested ANOVA design evaluates inter-method variability, while Bland-Altman plots assess agreement. Uncertainty budgets must include instrument precision, sample preparation, and calibration curve errors .
Q. How does this compound influence the pharmacodynamic activity of Lesinurad as a URAT1 inhibitor?
- Methodological Answer : Competitive binding assays (e.g., surface plasmon resonance) measure Impurity C’s affinity for URAT1 compared to Lesinurad. IC50 values derived from dose-response curves in HEK293 cells expressing URAT1 reveal whether Impurity C acts as an antagonist. Structural-activity relationship (SAR) analysis of Component C modifications (e.g., sulfhydryl group substitution) identifies critical pharmacophoric elements .
Q. Methodological and Ethical Considerations
Q. What criteria should guide the selection of impurity limits for this compound in regulatory submissions?
- Methodological Answer : Limits are based on ICH Q3A/B thresholds (e.g., 0.10% for daily doses ≤2 g/day). Justification requires toxicological risk assessment (Ames test, micronucleus assay) and process capability data (e.g., 6σ control charts). If Impurity C is a genotoxic suspect, a threshold of toxicological concern (TTC; ≤1.5 μg/day) applies .
Q. How can researchers ensure reproducibility in impurity profiling studies for this compound?
- Methodological Answer : Detailed method documentation (column lot numbers, mobile phase preparation protocols) and raw data archiving (e.g., electronic lab notebooks) are critical. Inter-laboratory studies using shared reference standards validate method transferability. Compliance with ALCOA+ principles (Attributable, Legible, Contemporaneous, Original, Accurate) ensures data integrity .
Q. Tables for Key Data
Parameter | HPLC-UV Method | LC-MS/MS Method |
---|---|---|
LOQ | 0.05% | 0.01% |
Linearity (R²) | 0.998 | 0.999 |
Recovery (%) | 98–102 | 95–105 |
Column | C18 (4.6 × 150 mm, 3 µm) | HILIC (2.1 × 100 mm, 1.7 µm) |
Reference |
Propriétés
IUPAC Name |
2-[(4-naphthalen-1-yl-1,2,4-triazol-3-yl)sulfanyl]acetic acid | |
---|---|---|
Source | PubChem | |
URL | https://pubchem.ncbi.nlm.nih.gov | |
Description | Data deposited in or computed by PubChem | |
InChI |
InChI=1S/C14H11N3O2S/c18-13(19)8-20-14-16-15-9-17(14)12-7-3-5-10-4-1-2-6-11(10)12/h1-7,9H,8H2,(H,18,19) | |
Source | PubChem | |
URL | https://pubchem.ncbi.nlm.nih.gov | |
Description | Data deposited in or computed by PubChem | |
InChI Key |
MSEBOVZSWIYSMG-UHFFFAOYSA-N | |
Source | PubChem | |
URL | https://pubchem.ncbi.nlm.nih.gov | |
Description | Data deposited in or computed by PubChem | |
Canonical SMILES |
C1=CC=C2C(=C1)C=CC=C2N3C=NN=C3SCC(=O)O | |
Source | PubChem | |
URL | https://pubchem.ncbi.nlm.nih.gov | |
Description | Data deposited in or computed by PubChem | |
Molecular Formula |
C14H11N3O2S | |
Source | PubChem | |
URL | https://pubchem.ncbi.nlm.nih.gov | |
Description | Data deposited in or computed by PubChem | |
Molecular Weight |
285.32 g/mol | |
Source | PubChem | |
URL | https://pubchem.ncbi.nlm.nih.gov | |
Description | Data deposited in or computed by PubChem | |
Retrosynthesis Analysis
AI-Powered Synthesis Planning: Our tool employs the Template_relevance Pistachio, Template_relevance Bkms_metabolic, Template_relevance Pistachio_ringbreaker, Template_relevance Reaxys, Template_relevance Reaxys_biocatalysis model, leveraging a vast database of chemical reactions to predict feasible synthetic routes.
One-Step Synthesis Focus: Specifically designed for one-step synthesis, it provides concise and direct routes for your target compounds, streamlining the synthesis process.
Accurate Predictions: Utilizing the extensive PISTACHIO, BKMS_METABOLIC, PISTACHIO_RINGBREAKER, REAXYS, REAXYS_BIOCATALYSIS database, our tool offers high-accuracy predictions, reflecting the latest in chemical research and data.
Strategy Settings
Precursor scoring | Relevance Heuristic |
---|---|
Min. plausibility | 0.01 |
Model | Template_relevance |
Template Set | Pistachio/Bkms_metabolic/Pistachio_ringbreaker/Reaxys/Reaxys_biocatalysis |
Top-N result to add to graph | 6 |
Feasible Synthetic Routes
Avertissement et informations sur les produits de recherche in vitro
Veuillez noter que tous les articles et informations sur les produits présentés sur BenchChem sont destinés uniquement à des fins informatives. Les produits disponibles à l'achat sur BenchChem sont spécifiquement conçus pour des études in vitro, qui sont réalisées en dehors des organismes vivants. Les études in vitro, dérivées du terme latin "in verre", impliquent des expériences réalisées dans des environnements de laboratoire contrôlés à l'aide de cellules ou de tissus. Il est important de noter que ces produits ne sont pas classés comme médicaments et n'ont pas reçu l'approbation de la FDA pour la prévention, le traitement ou la guérison de toute condition médicale, affection ou maladie. Nous devons souligner que toute forme d'introduction corporelle de ces produits chez les humains ou les animaux est strictement interdite par la loi. Il est essentiel de respecter ces directives pour assurer la conformité aux normes légales et éthiques en matière de recherche et d'expérimentation.