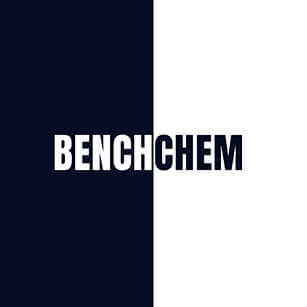
Ertapenem Dimer Amide Impurity
- Cliquez sur DEMANDE RAPIDE pour recevoir un devis de notre équipe d'experts.
- Avec des produits de qualité à un prix COMPÉTITIF, vous pouvez vous concentrer davantage sur votre recherche.
Vue d'ensemble
Description
Ertapenem Dimer Amide Impurity is a byproduct formed during the synthesis of Ertapenem, a carbapenem class antibiotic. Ertapenem is known for its broad antibacterial spectrum against Gram-positive and Gram-negative aerobes and anaerobes. The dimer impurities, including this compound, are identified and resolved using advanced chromatographic techniques .
Méthodes De Préparation
The preparation of Ertapenem Dimer Amide Impurity involves complex synthetic routes. The chromatographic separation of these impurities is achieved using high-performance liquid chromatography (HPLC) with gradient elution. The mobile phase typically consists of ammonium formate buffer, water, acetonitrile, and methanol at specific pH levels. The separation is highly dependent on the pH of the mobile phase, with optimal separation achieved at pH 8 .
Analyse Des Réactions Chimiques
Ertapenem Dimer Amide Impurity undergoes various chemical reactions, including oxidation, reduction, and substitution. Common reagents used in these reactions include ammonium formate buffer, acetonitrile, and methanol. The major products formed from these reactions are other dimer impurities and degradation products .
Applications De Recherche Scientifique
Ertapenem Dimer Amide Impurity is primarily studied in the context of pharmaceutical research to understand the stability and degradation pathways of Ertapenem. It is crucial for developing efficient methods to separate and identify impurities in drug formulations. This impurity profile helps in ensuring the safety and efficacy of the antibiotic .
Mécanisme D'action
Comparaison Avec Des Composés Similaires
Ertapenem Dimer Amide Impurity can be compared with other dimer impurities formed during the synthesis of carbapenem antibiotics. Similar compounds include Meropenem and Imipenem, which also form dimer impurities during their synthesis. The uniqueness of this compound lies in its specific formation conditions and the need for precise chromatographic techniques for its identification and separation .
Activité Biologique
Ertapenem is a member of the carbapenem class of antibiotics, known for its broad-spectrum antibacterial activity against various Gram-positive and Gram-negative bacteria. However, during the synthesis of Ertapenem, several impurities can form, including the Ertapenem Dimer Amide Impurity. Understanding the biological activity of this impurity is crucial for assessing the overall safety and efficacy of Ertapenem formulations.
Overview of this compound
This compound is a byproduct that occurs during the synthesis of Ertapenem. This compound is characterized by its unique chemical structure, which can influence its biological activity. The formation of such dimers is often linked to polymerization processes that can occur under specific conditions during drug synthesis and storage .
Antibacterial Spectrum
The biological activity of this compound has been evaluated in several studies. It has been noted that while Ertapenem effectively targets a wide range of bacteria, including those producing extended-spectrum beta-lactamases (ESBLs), the dimer impurity may not retain the same level of efficacy against all pathogens. For instance, it is generally ineffective against Pseudomonas aeruginosa and Acinetobacter baumannii, similar to Ertapenem itself .
Table 1: Antibacterial Activity Comparison
Pathogen | Ertapenem Activity | Dimer Amide Impurity Activity |
---|---|---|
Enterobacteriaceae | Active | Likely active |
Pseudomonas aeruginosa | Inactive | Inactive |
Acinetobacter baumannii | Inactive | Inactive |
Gram-positive bacteria | Active | Likely active |
Case Studies
Several clinical studies have assessed the implications of using Ertapenem in treating infections caused by ESBL-producing organisms. One study reported outcomes for patients treated with either Ertapenem or other carbapenems, highlighting that while Ertapenem was effective, there were concerns about the potential impact of impurities like the dimer amide on treatment outcomes. Specifically, patients treated with Ertapenem had lower rates of severe sepsis compared to those receiving other carbapenems .
Research Findings
Recent research has focused on the characterization and isolation of dimeric impurities from various carbapenems. A study indicated that dimer formation is influenced by concentration and storage conditions, which can affect the stability and efficacy of the antibiotic . Furthermore, chromatographic techniques such as high-performance liquid chromatography (HPLC) have been employed to separate and analyze these impurities effectively.
Table 2: Summary of Research Findings
Propriétés
Numéro CAS |
1199797-42-6 |
---|---|
Formule moléculaire |
C44H50N6O14S2 |
Poids moléculaire |
951.0 g/mol |
Nom IUPAC |
(4R,5S,6S)-3-[(3S,5S)-5-[[3-[(2S,3R)-5-carboxy-2-[(1S,2R)-1-carboxy-2-hydroxypropyl]-4-[(3S,5S)-5-[(3-carboxyphenyl)carbamoyl]pyrrolidin-3-yl]sulfanyl-3-methyl-2,3-dihydropyrrole-1-carbonyl]phenyl]carbamoyl]pyrrolidin-3-yl]sulfanyl-6-[(1R)-1-hydroxyethyl]-4-methyl-7-oxo-1-azabicyclo[3.2.0]hept-2-ene-2-carboxylic acid |
InChI |
InChI=1S/C44H50N6O14S2/c1-17-31-29(19(3)51)40(56)50(31)34(44(63)64)35(17)65-25-13-27(45-15-25)37(53)47-23-9-5-7-21(11-23)39(55)49-32(30(20(4)52)42(59)60)18(2)36(33(49)43(61)62)66-26-14-28(46-16-26)38(54)48-24-10-6-8-22(12-24)41(57)58/h5-12,17-20,25-32,45-46,51-52H,13-16H2,1-4H3,(H,47,53)(H,48,54)(H,57,58)(H,59,60)(H,61,62)(H,63,64)/t17-,18-,19-,20-,25+,26+,27+,28+,29-,30-,31-,32-/m1/s1 |
Clé InChI |
ATXZKQUYOZAYTG-BNCIILEKSA-N |
SMILES isomérique |
C[C@@H]1[C@@H]2[C@H](C(=O)N2C(=C1S[C@H]3C[C@H](NC3)C(=O)NC4=CC=CC(=C4)C(=O)N5[C@H]([C@H](C(=C5C(=O)O)S[C@H]6C[C@H](NC6)C(=O)NC7=CC=CC(=C7)C(=O)O)C)[C@@H]([C@@H](C)O)C(=O)O)C(=O)O)[C@@H](C)O |
SMILES canonique |
CC1C2C(C(=O)N2C(=C1SC3CC(NC3)C(=O)NC4=CC=CC(=C4)C(=O)N5C(C(C(=C5C(=O)O)SC6CC(NC6)C(=O)NC7=CC=CC(=C7)C(=O)O)C)C(C(C)O)C(=O)O)C(=O)O)C(C)O |
Apparence |
White Solid |
Pureté |
> 95% |
Quantité |
Milligrams-Grams |
Synonymes |
Ertapenem Dimer III; (4R,5S,6S)-3-[[(3S,5S)-5-[[[3-[[(2S,3R)-5-Carboxy-2-[(1S,2R)-1-carboxy-2-hydroxypropyl]-4-[[(3S,5S)-5-[[(3-carboxyphenyl)amino]carbonyl]-3-pyrrolidinyl]thio]-2,3-dihydro-3-methyl-1H-pyrrol-1-yl]carbonyl]phenyl]amino]carbonyl]-3-pyrr |
Origine du produit |
United States |
Avertissement et informations sur les produits de recherche in vitro
Veuillez noter que tous les articles et informations sur les produits présentés sur BenchChem sont destinés uniquement à des fins informatives. Les produits disponibles à l'achat sur BenchChem sont spécifiquement conçus pour des études in vitro, qui sont réalisées en dehors des organismes vivants. Les études in vitro, dérivées du terme latin "in verre", impliquent des expériences réalisées dans des environnements de laboratoire contrôlés à l'aide de cellules ou de tissus. Il est important de noter que ces produits ne sont pas classés comme médicaments et n'ont pas reçu l'approbation de la FDA pour la prévention, le traitement ou la guérison de toute condition médicale, affection ou maladie. Nous devons souligner que toute forme d'introduction corporelle de ces produits chez les humains ou les animaux est strictement interdite par la loi. Il est essentiel de respecter ces directives pour assurer la conformité aux normes légales et éthiques en matière de recherche et d'expérimentation.