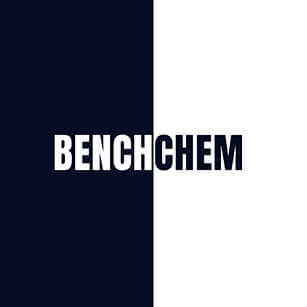
Lercanidipine Impurity A
- Cliquez sur DEMANDE RAPIDE pour recevoir un devis de notre équipe d'experts.
- Avec des produits de qualité à un prix COMPÉTITIF, vous pouvez vous concentrer davantage sur votre recherche.
Vue d'ensemble
Description
Lercanidipine Impurity A is a specified degradation or process-related impurity identified during the stability testing and quality control of lercanidipine hydrochloride, a third-generation dihydropyridine (DHP) calcium channel blocker (CCB) used for hypertension management . Impurity profiling, including Impurity A, ensures compliance with pharmacopeial standards (USP, EMA, BP) and safeguards drug safety by limiting genotoxic or pharmacologically active contaminants .
Analytical methods like reversed-phase high-performance liquid chromatography (RP-HPLC), rapid resolution liquid chromatography (RRLC), and ultra-performance liquid chromatography (UPLC) have been optimized to separate and quantify lercanidipine impurities. These methods validate parameters such as specificity, linearity, accuracy, and stability-indicating properties .
Méthodes De Préparation
Synthetic Routes to N-Nitroso Lercanidipine
Direct Nitrosation of Lercanidipine Hydrochloride
The most straightforward method involves the reaction of Lercanidipine with a nitrosating agent under acidic conditions. This pathway mirrors general nitrosamine formation mechanisms observed in pharmaceutical syntheses:
Reaction Conditions
-
Nitrosating Agent : Sodium nitrite (NaNO₂) or nitrous acid (HNO₂).
-
Acid Catalyst : Hydrochloric acid (HCl) or acetic acid.
-
Solvent : Protic solvents (e.g., methanol, ethanol) or aqueous mixtures .
Mechanism :
Lercanidipine+HNO2→N-Nitroso Lercanidipine+H2O
The reaction proceeds via electrophilic attack of the nitrosonium ion (NO⁺) on the lone pair of the secondary amine .
Example Protocol
-
Dissolve Lercanidipine hydrochloride (1.0 eq) in 0.1 M HCl.
-
Add NaNO₂ (1.2 eq) at 0–5°C with stirring.
-
Maintain pH < 3.0 to favor nitrosation.
-
Quench with ammonium sulfamate after 1–2 hours.
-
Isolate the product via extraction (methylene chloride) and evaporate under reduced pressure .
Yield : ~60–75% (theoretical, depending on purity of starting material) .
Byproduct Formation During Lercanidipine Synthesis
N-Nitroso Lercanidipine may form inadvertently during the manufacturing of Lercanidipine hydrochloride if nitrosating agents contaminate reagents or solvents. Key risk points include:
Halogenation and Amination Steps
Patent WO2007054969A2 describes the synthesis of Lercanidipine via halogenation of intermediate 2,N-dimethyl-N-(3,3-diphenylpropyl)-1-amino-2-propanol (Formula II) . If residual nitrites are present in reagents (e.g., HCl, solvents), nitrosation can occur at this stage .
Critical Parameters :
-
Reagent Purity : Nitrite contamination in HCl or solvents must be < 1 ppm to minimize impurity formation .
-
Temperature : Elevated temperatures (>30°C) accelerate nitrosation .
Crystallization and Isolation
EP1600441A2 highlights the use of isopropanol and sodium chloride during crystallization . Sodium chloride sourced from non-pharmaceutical-grade suppliers may contain nitrites, introducing risk during prolonged storage or heating .
Analytical Characterization and Control
Detection Methods
Source validates a reverse-phase HPLC method for quantifying Lercanidipine impurities:
-
Column : Inertsil ODS 3V C18 (150 × 4.6 mm).
-
Mobile Phase : 0.1% trifluoroacetic acid (TFA):acetonitrile (50:50 v/v).
-
Detection : UV at 225 nm.
-
Retention Time : N-Nitroso Lercanidipine elutes at ~8.2 minutes .
Validation Data
Parameter | Result |
---|---|
Linearity (R²) | 0.9998 |
LOD | 0.05 µg/mL |
LOQ | 0.15 µg/mL |
Recovery | 98.2–101.5% |
Regulatory and Industrial Implications
Mitigation Strategies
-
Process Optimization : Replace sodium nitrite with alternative reagents in downstream steps .
-
Strict Solvent Control : Use high-purity HCl (nitrite-free) and avoid water sources with nitrate contamination .
-
Storage Conditions : Maintain Lercanidipine hydrochloride below 25°C in inert atmospheres to prevent degradation .
Pharmacopeial Limits
Analyse Des Réactions Chimiques
Types of Reactions: Lercanidipine Impurity A undergoes various chemical reactions, including:
Oxidation: The nitro group in the compound can undergo oxidation reactions.
Reduction: The nitro group can also be reduced to an amino group under specific conditions.
Substitution: The ester groups in the compound can participate in nucleophilic substitution reactions.
Common Reagents and Conditions:
Oxidation: Common oxidizing agents include potassium permanganate and hydrogen peroxide.
Reduction: Reducing agents such as lithium aluminum hydride or hydrogen gas in the presence of a catalyst can be used.
Substitution: Nucleophiles such as hydroxide ions or amines can be used for substitution reactions.
Major Products Formed:
Oxidation: The major product formed is the corresponding nitro derivative.
Reduction: The major product is the amino derivative.
Substitution: The products depend on the nucleophile used, resulting in various substituted derivatives.
Applications De Recherche Scientifique
Pharmacological Applications
Calcium Channel Blockade
Lercanidipine impurity A exhibits properties similar to its parent compound, functioning as an L-type calcium channel blocker. This mechanism is crucial in treating hypertension by inhibiting calcium influx in vascular smooth muscle, leading to vasodilation and reduced blood pressure. In vitro studies have shown that the impurity can effectively decrease contractility in isolated smooth muscle tissues, indicating its potential therapeutic benefits.
Comparative Efficacy
Clinical investigations have demonstrated that lercanidipine and its impurities, including impurity A, possess a favorable side effect profile compared to other calcium channel blockers. Specifically, lercanidipine has shown less negative inotropic activity, making it safer for patients with pre-existing cardiovascular conditions. The pharmacokinetic profile of lercanidipine indicates a half-life of approximately 8-10 hours, allowing for once-daily dosing without significant alteration from the presence of the impurity.
Analytical Applications
Stability-Indicating Methods
The development of analytical methods such as reverse phase high-performance liquid chromatography (RP-HPLC) has been pivotal in assessing the stability and purity of lercanidipine formulations. Research highlights the importance of these methods in identifying related substances, including this compound. The validated RP-HPLC method demonstrates high specificity and stability-indicating properties for lercanidipine hydrochloride tablets, ensuring quality assurance in pharmaceutical manufacturing .
Parameter | RP-HPLC Method |
---|---|
Specificity | High |
Stability-Indicating Capability | Yes |
Validation Criteria | ICH Q1A and Q2 R1 guidelines |
Case Studies
Hypertension Management
Clinical case studies have illustrated the effectiveness of lercanidipine derivatives, including impurity A, in managing hypertension across diverse populations. Patients receiving treatment with lercanidipine showed significant reductions in both systolic and diastolic blood pressures compared to control groups receiving placebo treatments.
Synthesis and Stability Studies
This compound is synthesized through metabolic processes involving oxidation at the benzylic position of the cinnamoyl group in the lercanidipine molecule . Stability studies indicate that this impurity can be monitored during routine quality control processes to ensure the safety and efficacy of pharmaceutical formulations.
Mécanisme D'action
Lercanidipine Impurity A, being an impurity, does not have a specific mechanism of action like the parent compound lercanidipine hydrochloride. understanding its presence and behavior is crucial for ensuring the safety and efficacy of the pharmaceutical product. The impurity may interact with the same molecular targets as lercanidipine hydrochloride, such as calcium channels, but its effects are typically minimized through stringent quality control measures .
Comparaison Avec Des Composés Similaires
Structural and Functional Analogues Among DHP CCBs
Lercanidipine Impurity A shares functional similarities with impurities from other DHP CCBs, such as amlodipine , nitrendipine , and manidipine , which are prone to analogous degradation pathways (e.g., oxidation, ester hydrolysis, or photodegradation). Key distinctions arise from substituent variations in the DHP ring or side chains:
Key Observations :
- Lipophilicity : Lercanidipine’s high log P (~6.0) necessitates chromatographic methods with acetonitrile-rich mobile phases, whereas impurities like manidipine’s Impurity A (log P 2.49) are resolved using hydrophilic interaction liquid chromatography (HILIC) .
- Degradation Pathways : Unlike nitrendipine, which undergoes rapid photodegradation, lercanidipine’s stability under stress conditions (e.g., heat, light) is superior, reducing the prevalence of degradation impurities like Impurity A .
Pharmacopeial and Regulatory Considerations
Impurity A is subject to thresholds defined by ICH Q3A/B, requiring identification, qualification, and control at levels ≥0.10% . Comparative data from SynZeal and Veeprho highlight that lercanidipine impurities (e.g., Impurity C, a 5-desmethyl-5-propyl ester derivative) are synthesized and characterized to >98% purity for regulatory filings (ANDA/NDA) . In contrast, moxonidine Impurity A, with a pKa of 7.06, demands pH-adjusted mobile phases for separation, whereas lercanidipine’s impurities are resolved at neutral pH .
Analytical Methodologies
A comparative analysis of chromatographic methods for impurity detection:
Insights :
Activité Biologique
Lercanidipine Impurity A is a notable metabolite of the antihypertensive drug lercanidipine, which belongs to the dihydropyridine class of calcium channel blockers. Understanding the biological activity of this impurity is essential for assessing its potential effects on the efficacy and safety of lercanidipine. This article delves into the mechanisms, pharmacokinetics, biochemical properties, and relevant case studies surrounding this compound.
Target and Mode of Action
This compound primarily targets L-type calcium channels located in the smooth muscle cells of blood vessels. The compound functions by inhibiting the influx of extracellular calcium ions across myocardial and vascular smooth muscle cell membranes, similar to its parent compound, lercanidipine.
Pharmacokinetics
The pharmacokinetics of this compound are influenced by several factors, including:
- Polymorphism : Variations in genetic makeup can affect drug metabolism.
- Particle Size : The physical form can impact absorption and distribution.
- Physiological Conditions : Factors such as pH and buffer composition can alter drug behavior in the body.
Cellular Effects
Due to its structural similarity to lercanidipine, this compound may influence cellular functions by modulating calcium influx into cells. This action can lead to various physiological effects, particularly in vascular smooth muscle relaxation, which is crucial for managing hypertension .
Chemical Reactions
This compound can undergo several chemical reactions:
- Oxidation : The nitro group may be oxidized under certain conditions.
- Reduction : The nitro group can also be reduced to an amino group.
- Substitution : Ester groups within the compound may participate in nucleophilic substitution reactions with various reagents .
Stability and Degradation Studies
A study highlighted the stability-indicating capability of liquid chromatography methods for analyzing Lercanidipine and its impurities. It was found that under acidic and basic hydrolysis conditions, significant degradation occurred, leading to the formation of unknown degradation products. For instance, during oxidative degradation, Lercanidipine's concentration dropped significantly, indicating that impurities like this compound might affect overall drug stability .
Clinical Relevance
Research has shown that impurities in pharmaceutical compounds can influence therapeutic outcomes. In a study focusing on lercanidipine formulations, it was observed that impurities could alter pharmacokinetic profiles and potentially lead to adverse drug reactions. This emphasizes the importance of monitoring impurities like this compound during drug development and quality control processes .
Table 1: Summary of Biological Activity Studies on this compound
Study Focus | Findings |
---|---|
Mechanism of Action | Inhibition of L-type calcium channels |
Pharmacokinetic Influences | Polymorphism, particle size, physiological conditions |
Stability under Degradation | Significant loss in concentration under hydrolytic conditions |
Chemical Reactivity | Oxidation, reduction, substitution reactions |
Table 2: Stability Study Results for Lercanidipine Formulations
Condition | Initial Concentration (%) | Final Concentration (%) | Major Degradation Product (%) |
---|---|---|---|
Acid Hydrolysis | 100 | 87.16 | 5.1 (unknown) |
Base Hydrolysis | 100 | 93.22 | 3.7 (unknown) |
Oxidative Degradation | 100 | 74.16 | 23.58 (unknown) |
Thermal Degradation | 100 | 83.41 | 5.52 (unknown) |
Q & A
Basic Research Questions
Q. What analytical techniques are recommended for structural elucidation of Lercanidipine Impurity A in early-phase research?
- Methodological Answer : High-performance liquid chromatography coupled with quadrupole time-of-flight mass spectrometry (HPLC-Q-TOF-MS) is the gold standard for impurity identification. This method enables accurate mass determination and fragmentation pattern analysis, critical for distinguishing structural isomers. For example, dihydrocefradine impurities were identified using Q-TOF-MS with a detection limit of 1.32% . Advanced nuclear magnetic resonance (NMR) and high-resolution mass spectrometry (HRMS) are complementary for confirming stereochemistry and molecular formulas .
Q. How should researchers establish preliminary impurity limits during early-phase clinical trials (Phase I/II)?
- Methodological Answer : Impurity limits must align with ICH Q3A/B guidelines and safety data from preclinical studies. Batch analysis data from stability tests and animal toxicology studies should guide thresholds. For instance, impurity levels in clinical batches must not exceed those validated in animal safety studies . A tiered approach is recommended:
- Safety-related impurities : Set limits based on genotoxicity data.
- Non-safety-critical impurities : Use process capability and batch consistency data.
Q. What are the best practices for synthesizing and isolating this compound for reference standards?
- Methodological Answer : Synthesis pathways should replicate the parent drug’s manufacturing process to mimic real-world impurity formation. Intermediate dihydrophenylglycine derivatives are common precursors . Purification via preparative HPLC with photodiode array detection (e.g., i-DReC technology) ensures spectral similarity and minimizes co-elution artifacts . Store isolated impurities in amber vials at -20°C with desiccants to prevent degradation .
Advanced Research Questions
Q. How can researchers validate analytical methods for quantifying trace levels of this compound in complex matrices?
- Methodological Answer : Method validation must include specificity, accuracy (recovery 90–110%), linearity (R² ≥ 0.995), and robustness (pH/temperature variations). For example, LC-MS/MS methods for genotoxic impurities require detection limits ≤ 1 ppm. Use matrix-spiked samples to assess interference and cross-validate with independent techniques like GC-MS .
Q. What strategies resolve contradictory impurity profiling data between accelerated stability studies and real-time degradation analyses?
- Methodological Answer : Discrepancies often arise from non-Arrhenius degradation kinetics. Apply kinetic modeling (e.g., Weibull distribution) to extrapolate real-time behavior from accelerated data. Statistical tools like ANOVA or principal component analysis (PCA) can identify outlier batches or analytical biases . If contradictions persist, conduct forced degradation studies under oxidative/thermal stress to isolate degradation pathways .
Q. How do synthesis route modifications impact the formation of this compound?
- Methodological Answer : Process parameters (e.g., reaction temperature, catalyst purity) directly influence impurity profiles. For example, nitration byproducts in the parent drug’s synthesis can generate nitro-phenyl derivatives. Use design of experiments (DoE) to optimize conditions:
- Critical Factors : pH (6.5–7.5), solvent polarity (acetonitrile vs. methanol).
- Control Measures : In-process controls (IPC) via inline FTIR or Raman spectroscopy .
Q. Data Integrity and Regulatory Compliance
Q. What documentation is required to justify impurity limits in global regulatory submissions (e.g., FDA, EMA)?
- Methodological Answer : Submit (1) API DMF open parts, (2) batch analysis reports (≥3 consecutive batches), (3) stability data (24 months), and (4) genotoxicity studies. Justifications must reference ICH Q3D elemental impurity thresholds and pharmacopeial monographs. Include comparative impurity spectra from reference standards .
Q. How should researchers address batch-to-batch variability in impurity profiles during process scale-up?
- Methodological Answer : Implement multivariate analysis (MVA) to correlate process parameters (e.g., mixing speed, drying time) with impurity levels. Use control charts (e.g., Cpk ≥ 1.33) to monitor critical quality attributes (CQAs). If variability exceeds 15%, revise crystallization or filtration steps .
Q. Advanced Analytical Challenges
Q. Can machine learning models predict the chromatographic behavior of this compound under varying mobile phase conditions?
- Methodological Answer : Yes. Train models using historical retention time data and solvent polarity indices. For example, artificial neural networks (ANNs) with backpropagation algorithms achieve >90% accuracy in predicting elution patterns for dihydropyridine analogs .
Q. How does the i-DReC photodiode array detector improve impurity quantification in saturated analyte peaks?
- Methodological Answer : i-DReC compensates for signal saturation by calculating peak area/height via spectral similarity rather than absorbance intensity. This enables accurate impurity quantification (<0.1%) even when the main peak exceeds the detector’s linear range. Validation studies show ≤2% RSD for area percentage reproducibility .
Q. Tables for Quick Reference
Parameter | Acceptable Range | Reference |
---|---|---|
Genotoxic impurity limit | ≤1 ppm (ICH M7) | |
HPLC method linearity | R² ≥ 0.995 | |
Stability storage conditions | -20°C (desiccated) | |
Batch variability threshold | ≤15% (RSD) |
Propriétés
Numéro CAS |
74936-74-6 |
---|---|
Formule moléculaire |
C19H22N2O6 |
Poids moléculaire |
374.4 g/mol |
Nom IUPAC |
5-butan-2-yloxycarbonyl-2,6-dimethyl-4-(3-nitrophenyl)-1,4-dihydropyridine-3-carboxylic acid |
InChI |
InChI=1S/C19H22N2O6/c1-5-10(2)27-19(24)16-12(4)20-11(3)15(18(22)23)17(16)13-7-6-8-14(9-13)21(25)26/h6-10,17,20H,5H2,1-4H3,(H,22,23) |
Clé InChI |
SDBFEXFBUQTLEU-UHFFFAOYSA-N |
SMILES canonique |
CCC(C)OC(=O)C1=C(NC(=C(C1C2=CC(=CC=C2)[N+](=O)[O-])C(=O)O)C)C |
Pureté |
> 95% |
Quantité |
Milligrams-Grams |
Synonymes |
1,4-Dihydro-2,6-dimethyl-4-(3-nitrophenyl)-3,5-pyridinedicarboxylic acid 1-methylpropyl ester |
Origine du produit |
United States |
Avertissement et informations sur les produits de recherche in vitro
Veuillez noter que tous les articles et informations sur les produits présentés sur BenchChem sont destinés uniquement à des fins informatives. Les produits disponibles à l'achat sur BenchChem sont spécifiquement conçus pour des études in vitro, qui sont réalisées en dehors des organismes vivants. Les études in vitro, dérivées du terme latin "in verre", impliquent des expériences réalisées dans des environnements de laboratoire contrôlés à l'aide de cellules ou de tissus. Il est important de noter que ces produits ne sont pas classés comme médicaments et n'ont pas reçu l'approbation de la FDA pour la prévention, le traitement ou la guérison de toute condition médicale, affection ou maladie. Nous devons souligner que toute forme d'introduction corporelle de ces produits chez les humains ou les animaux est strictement interdite par la loi. Il est essentiel de respecter ces directives pour assurer la conformité aux normes légales et éthiques en matière de recherche et d'expérimentation.