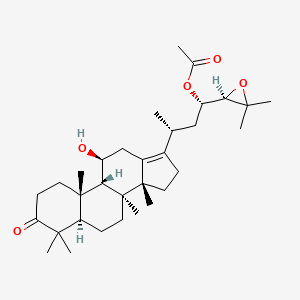
Alisol B 23-acetate
Vue d'ensemble
Description
Alisol B 23-acetate (AB23A) is a natural triterpenoid, which is a major bioactive constituent in the Chinese herb Zexie (Rhizoma Alismatis) . It has been found to have multiple pharmacological activities . AB23A produces protective effects against EE-induced cholestasis due to FXR-mediated gene regulation .
Synthesis Analysis
AB23A can be readily hydrolyzed to form alisol B in human plasma, intestinal, and hepatic preparations . The key enzymes responsible for AB23A hydrolysis in human plasma and tissue preparations are human butyrylcholinesterase (hBchE) and human carboxylesterases . It was also found that human serum albumin (hSA) could catalyze AB23A hydrolysis .Molecular Structure Analysis
The molecular formula of AB23A is C32H50O5 . Its molecular weight is 514.74 .Chemical Reactions Analysis
AB23A can be readily hydrolyzed to form alisol B in human plasma, intestinal, and hepatic preparations . The key enzymes responsible for AB23A hydrolysis in human plasma and tissue preparations are human butyrylcholinesterase (hBchE) and human carboxylesterases .Physical And Chemical Properties Analysis
AB23A is a solid substance with a white to off-white color . It has a molecular weight of 514.74 and a molecular formula of C32H50O5 .Applications De Recherche Scientifique
Anti-Inflammatory Activity
AB23A, a natural triterpenoid, has been reported to exert anti-inflammatory activity . It inhibits the degranulation of mast cells stimulated by immunoglobulin E/antigen (IgE/Ag), and also decreases the synthesis of leukotriene C4 (LTC4), production of interlukin-6 (IL-6), and expression of cyclooxygenase-2 (COX-2) in a concentration-dependent manner . This suggests that AB23A inhibits the activation of mast cells and ameliorates allergic reaction .
Hepatoprotective Activity
AB23A has been reported to exert hepatoprotective activities . However, the specific mechanisms and applications in this field require further investigation.
Antitumor Activities
AB23A has been reported to exert anti-proliferative activities in human colon, ovarian, and gastric cancer cells . It activates the apoptosis-associated proteins, suggesting its potential as a therapeutic agent in cancer treatment .
Cardiovascular Disease Treatment
Research suggests that AB23A can effectively treat cardiovascular diseases by regulating the lipid metabolism of macrophages . It improves the biological behavior of vascular smooth muscle cells (VSMCs) and enhances anti-inflammatory effects .
Metabolic Pathway Analysis
AB23A can be readily hydrolyzed to form alisol B in human plasma, intestinal, and hepatic preparations . Human butyrylcholinesterase (hBchE) and human carboxylesterases play key roles in AB23A hydrolysis in human plasma and tissue preparations, respectively . This study is very helpful for understanding the metabolic rates of AB23A in humans .
FXR Agonist Effect
Both AB23A and alisol B exhibit similar FXR agonist effects, indicating AB23A hydrolysis does not affect its FXR agonist effect . This could have implications for the development of novel prodrugs of alisol B with desirable pharmacokinetic behaviors .
Mécanisme D'action
Target of Action
Alisol B 23-acetate (AB23A) is a natural triterpenoid that primarily targets the Farnesoid X receptor (FXR) . FXR plays a pivotal role in regulating renal function and is involved in the regulation of genes involved in hepatocyte proliferation .
Mode of Action
AB23A activates FXR, leading to the regulation of various downstream genes . It also interacts with human butyrylcholinesterase (hBchE) and human carboxylesterases, key enzymes responsible for AB23A hydrolysis in human plasma and tissue preparations, respectively .
Biochemical Pathways
AB23A affects several biochemical pathways. It inhibits the TLR4-NOX1/ROS signaling pathway in Caco-2 cells . It also regulates the expression of ATP-binding cassette transfer proteins G5/G8 (ABCG5/G8) and the liver X receptor α (LXRα) . Furthermore, it influences the regulation of transporters and enzymes involved in bile acid homeostasis .
Pharmacokinetics
AB23A can be readily hydrolyzed to form alisol B in human plasma, intestinal, and hepatic preparations . The hydrolytic pathways of AB23A in humans involve key enzymes such as hBchE and human carboxylesterases .
Result of Action
The activation of FXR by AB23A exerts renoprotective action in animals with acute kidney injury (AKI) . It also ameliorates lipopolysaccharide-induced intestinal barrier dysfunction by inhibiting TLR4-NOX1/ROS signaling pathway in Caco-2 cells . Moreover, it protects against ANIT-induced hepatotoxicity and cholestasis .
Action Environment
The action of AB23A is influenced by various environmental factors. For instance, the hydrolytic pathways of AB23A in human biological systems help in understanding the metabolic rates of AB23A in humans . The protective effects of AB23A are FXR-dependent . Therefore, the presence or absence of FXR in the environment significantly influences the compound’s action, efficacy, and stability.
Safety and Hazards
Orientations Futures
Propriétés
IUPAC Name |
[(1S,3R)-1-[(2R)-3,3-dimethyloxiran-2-yl]-3-[(5R,8S,9S,10S,11S,14R)-11-hydroxy-4,4,8,10,14-pentamethyl-3-oxo-1,2,5,6,7,9,11,12,15,16-decahydrocyclopenta[a]phenanthren-17-yl]butyl] acetate | |
---|---|---|
Source | PubChem | |
URL | https://pubchem.ncbi.nlm.nih.gov | |
Description | Data deposited in or computed by PubChem | |
InChI |
InChI=1S/C32H50O5/c1-18(16-23(36-19(2)33)27-29(5,6)37-27)20-10-14-31(8)21(20)17-22(34)26-30(7)13-12-25(35)28(3,4)24(30)11-15-32(26,31)9/h18,22-24,26-27,34H,10-17H2,1-9H3/t18-,22+,23+,24+,26+,27-,30+,31+,32+/m1/s1 | |
Source | PubChem | |
URL | https://pubchem.ncbi.nlm.nih.gov | |
Description | Data deposited in or computed by PubChem | |
InChI Key |
NLOAQXKIIGTTRE-JSWHPQHOSA-N | |
Source | PubChem | |
URL | https://pubchem.ncbi.nlm.nih.gov | |
Description | Data deposited in or computed by PubChem | |
Canonical SMILES |
CC(CC(C1C(O1)(C)C)OC(=O)C)C2=C3CC(C4C5(CCC(=O)C(C5CCC4(C3(CC2)C)C)(C)C)C)O | |
Source | PubChem | |
URL | https://pubchem.ncbi.nlm.nih.gov | |
Description | Data deposited in or computed by PubChem | |
Isomeric SMILES |
C[C@H](C[C@@H]([C@@H]1C(O1)(C)C)OC(=O)C)C2=C3C[C@@H]([C@H]4[C@]5(CCC(=O)C([C@@H]5CC[C@@]4([C@]3(CC2)C)C)(C)C)C)O | |
Source | PubChem | |
URL | https://pubchem.ncbi.nlm.nih.gov | |
Description | Data deposited in or computed by PubChem | |
Molecular Formula |
C32H50O5 | |
Source | PubChem | |
URL | https://pubchem.ncbi.nlm.nih.gov | |
Description | Data deposited in or computed by PubChem | |
Molecular Weight |
514.7 g/mol | |
Source | PubChem | |
URL | https://pubchem.ncbi.nlm.nih.gov | |
Description | Data deposited in or computed by PubChem | |
CAS RN |
26575-95-1 | |
Record name | ALISOL B 23-ACETATE | |
Source | FDA Global Substance Registration System (GSRS) | |
URL | https://gsrs.ncats.nih.gov/ginas/app/beta/substances/72Y3NE3ZV8 | |
Description | The FDA Global Substance Registration System (GSRS) enables the efficient and accurate exchange of information on what substances are in regulated products. Instead of relying on names, which vary across regulatory domains, countries, and regions, the GSRS knowledge base makes it possible for substances to be defined by standardized, scientific descriptions. | |
Explanation | Unless otherwise noted, the contents of the FDA website (www.fda.gov), both text and graphics, are not copyrighted. They are in the public domain and may be republished, reprinted and otherwise used freely by anyone without the need to obtain permission from FDA. Credit to the U.S. Food and Drug Administration as the source is appreciated but not required. | |
Q & A
Q1: What are the primary molecular targets of Alisol B 23-acetate?
A1: Research suggests that AB23A interacts with multiple molecular targets, contributing to its pleiotropic effects. Some of the identified targets include:
- Farnesoid X receptor (FXR): AB23A acts as an agonist of FXR [], a nuclear receptor crucial for regulating bile acid, lipid, and glucose homeostasis. This interaction has implications for its hepatoprotective and anti-atherosclerotic effects [, ].
- Spleen tyrosine kinase (Syk): AB23A demonstrates inhibitory effects on Syk [], a key mediator of immune receptor signaling in mast cells. This interaction likely contributes to its anti-allergic and anti-inflammatory properties [, ].
- mTOR signaling pathway: Studies indicate that AB23A inhibits the mTOR pathway [], a central regulator of cell growth, proliferation, and survival. This interaction holds promise for its anticancer activity, particularly in liver cancer [].
- Wnt/β-catenin pathway: AB23A influences the Wnt/β-catenin signaling pathway [, ], involved in cell fate determination, proliferation, and differentiation. This interaction may play a role in its anticancer effects in various cancers, including liver and colon cancer [, ].
Q2: How does Alisol B 23-acetate's interaction with FXR contribute to its hepatoprotective effects?
A2: By activating FXR, AB23A influences several processes that contribute to liver protection:
- Reduced hepatic bile acid accumulation: AB23A, via FXR activation, downregulates the expression of the bile acid uptake transporter (Ntcp) and bile acid synthesis enzymes (Cyp7a1 and Cyp8b1), while upregulating the bile acid efflux transporter (Bsep and Mrp2) []. This coordinated regulation helps to decrease bile acid levels in the liver, protecting against bile acid-induced toxicity.
- Enhanced hepatocyte proliferation: AB23A, through FXR activation, increases the expression of FoxM1b, Cyclin D1, and Cyclin B1, promoting liver regeneration and recovery from injury [].
Q3: What are the downstream consequences of Alisol B 23-acetate inhibiting the mTOR pathway in cancer cells?
A3: Inhibition of the mTOR pathway by AB23A triggers a cascade of events leading to:
- Disruption of ribosomal biogenesis: AB23A interferes with the mTOR-MRP axis, which plays a critical role in ribosome biogenesis, essential for protein synthesis and cell growth []. This disruption hampers the rapid proliferation characteristic of cancer cells.
- G1 cell cycle arrest: By inhibiting mTOR, AB23A arrests the cell cycle at the G1 phase, preventing DNA replication and cell division [].
- Induction of apoptosis: AB23A's inhibition of mTOR ultimately leads to the activation of apoptotic pathways, culminating in programmed cell death [, ].
Q4: What is the molecular formula and weight of Alisol B 23-acetate?
A4: Alisol B 23-acetate has the molecular formula C32H50O5 and a molecular weight of 514.74 g/mol.
Q5: What in vitro models have been used to study the effects of Alisol B 23-acetate?
A5: Various cell lines have been employed to investigate the effects of AB23A in vitro, including:
- HepG2 cells: This human liver cancer cell line is widely used to study AB23A's anti-cancer activity, particularly its effects on cell viability, apoptosis, and the mTOR pathway [].
- HCT116 cells: This human colon cancer cell line has been utilized to investigate AB23A's ability to induce autophagy, cell cycle arrest, and apoptosis [].
- RBL-2H3 cells and BMMCs: These mast cell lines, along with primary bone marrow-derived mast cells (BMMCs), are commonly used to study AB23A's anti-allergic effects, focusing on its ability to inhibit degranulation and inflammatory mediator release [, ].
- Caco-2 cells: This human intestinal epithelial cell line has been used to investigate AB23A's effects on intestinal barrier function, particularly its ability to modulate tight junction proteins and reduce permeability [].
Q6: What in vivo models have been used to study the effects of Alisol B 23-acetate?
A6: Several animal models have been employed to investigate the effects of AB23A in vivo:
- Rodent models of allergic asthma: Ovalbumin-induced allergic asthma models in mice are frequently used to evaluate AB23A's anti-allergic and anti-inflammatory effects in the airways [].
- Rodent models of non-alcoholic fatty liver disease (NAFLD): Mice fed with a methionine and choline-deficient (MCD) diet are used to induce NASH, allowing researchers to investigate the hepatoprotective effects of AB23A and its impact on lipid metabolism, oxidative stress, and inflammation [].
- Rodent models of chronic kidney disease (CKD): 5/6 nephrectomised (NX) and unilateral ureteral obstructed (UUO) rat models are commonly used to study the renoprotective effects of AB23A in CKD, focusing on its ability to reduce renal fibrosis, inflammation, and improve renal function [].
- Rodent models of atherosclerosis: ApoE-/- mice fed with a high-fat diet are frequently used to induce atherosclerosis. These models allow for the evaluation of AB23A's lipid-lowering and anti-atherosclerotic effects, as well as its impact on cholesterol metabolism and plaque formation [].
Q7: Have there been any clinical trials investigating the effects of Alisol B 23-acetate in humans?
A7: While preclinical studies have demonstrated promising results, there are currently no published clinical trials investigating the safety and efficacy of AB23A in humans. Further research, including well-designed clinical trials, is necessary to translate these findings into clinical practice.
Q8: What analytical techniques are commonly used to quantify Alisol B 23-acetate in plant materials and pharmaceutical formulations?
A8: Several analytical methods have been developed and validated for the quantification of AB23A, including:
- High-Performance Liquid Chromatography (HPLC): HPLC, coupled with various detectors like ultraviolet (UV), diode-array detection (DAD), and evaporative light scattering detection (ELSD), is widely employed for the separation and quantification of AB23A in complex matrices [, , , , , , , , , ].
- Ultra-High Performance Liquid Chromatography (UHPLC): UHPLC, offering enhanced resolution and sensitivity compared to conventional HPLC, is increasingly used for the analysis of AB23A, particularly in combination with mass spectrometry (MS) for accurate identification and quantification [, , ].
- Thin-Layer Chromatography (TLC): TLC, a simpler and more rapid technique, is often employed for the preliminary identification and screening of AB23A in plant materials and formulations [, ].
Q9: What are the key considerations for ensuring the quality control of Alisol B 23-acetate in pharmaceutical products?
A9: Maintaining consistent quality and efficacy of AB23A-containing products requires stringent quality control measures throughout the production process:
- Standardization of raw materials: Ensuring the quality and consistency of Alisma orientale rhizome used for extraction is crucial. This involves controlling factors like plant origin, harvesting time, and drying conditions, as these can impact the content of AB23A and other bioactive compounds [, ].
- Optimization of extraction and purification methods: Selecting appropriate extraction solvents and optimizing extraction parameters like temperature, time, and solvent-to-sample ratio are critical for maximizing AB23A yield while minimizing the co-extraction of unwanted compounds [, ].
- Establishment of validated analytical methods: Employing validated analytical methods, such as HPLC or UHPLC, with appropriate sensitivity, accuracy, precision, and specificity is crucial for quantifying AB23A content and ensuring batch-to-batch consistency in final products [, , , , ].
- Stability studies: Investigating the stability of AB23A under various storage conditions (temperature, humidity, light exposure) is vital for determining appropriate packaging, shelf life, and storage recommendations to maintain product quality over time [, ].
Avertissement et informations sur les produits de recherche in vitro
Veuillez noter que tous les articles et informations sur les produits présentés sur BenchChem sont destinés uniquement à des fins informatives. Les produits disponibles à l'achat sur BenchChem sont spécifiquement conçus pour des études in vitro, qui sont réalisées en dehors des organismes vivants. Les études in vitro, dérivées du terme latin "in verre", impliquent des expériences réalisées dans des environnements de laboratoire contrôlés à l'aide de cellules ou de tissus. Il est important de noter que ces produits ne sont pas classés comme médicaments et n'ont pas reçu l'approbation de la FDA pour la prévention, le traitement ou la guérison de toute condition médicale, affection ou maladie. Nous devons souligner que toute forme d'introduction corporelle de ces produits chez les humains ou les animaux est strictement interdite par la loi. Il est essentiel de respecter ces directives pour assurer la conformité aux normes légales et éthiques en matière de recherche et d'expérimentation.