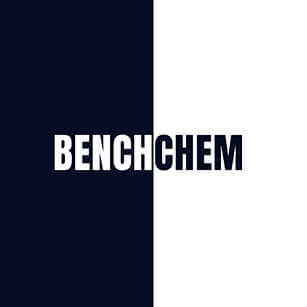
HIV (GP120) ANTIGENIC PEPTIDE
- Cliquez sur DEMANDE RAPIDE pour recevoir un devis de notre équipe d'experts.
- Avec des produits de qualité à un prix COMPÉTITIF, vous pouvez vous concentrer davantage sur votre recherche.
Vue d'ensemble
Description
The HIV (GP120) antigenic peptide is a crucial component of the Human Immunodeficiency Virus (HIV) envelope glycoprotein complex. This peptide plays a significant role in the virus’s ability to infect host cells. The GP120 subunit, derived from the precursor glycoprotein GP160, is essential for the initial binding of the virus to the host cell receptors, primarily the CD4 receptor and co-receptors such as CCR5 and CXCR4 . This interaction facilitates the fusion of the viral and host cell membranes, allowing the virus to enter and infect the host cell .
Méthodes De Préparation
Synthetic Routes and Reaction Conditions: The synthesis of the HIV (GP120) antigenic peptide typically involves recombinant DNA technology. The gene encoding the GP120 protein is inserted into an expression vector, which is then introduced into a suitable host cell, such as Escherichia coli or mammalian cells . The host cells are cultured under specific conditions to express the GP120 protein, which is subsequently purified using chromatographic techniques .
Industrial Production Methods: Industrial production of the this compound follows similar principles but on a larger scale. Bioreactors are used to culture the host cells, and advanced purification methods, including affinity chromatography and high-performance liquid chromatography, are employed to ensure the purity and quality of the peptide .
Analyse Des Réactions Chimiques
Types of Reactions: The HIV (GP120) antigenic peptide undergoes various chemical reactions, including glycosylation, which is crucial for its proper folding and function . Glycosylation involves the addition of carbohydrate moieties to specific amino acid residues, which can affect the peptide’s antigenicity and immunogenicity .
Common Reagents and Conditions: Common reagents used in the glycosylation of the GP120 peptide include glycosyltransferases and nucleotide sugars . The reactions typically occur in the endoplasmic reticulum and Golgi apparatus of the host cells under physiological conditions .
Major Products Formed: The major products formed from these reactions are glycosylated forms of the GP120 peptide, which are essential for its interaction with host cell receptors and subsequent viral entry .
Applications De Recherche Scientifique
The HIV (GP120) antigenic peptide has numerous applications in scientific research:
Vaccine Development: The GP120 peptide is a key target for HIV vaccine development due to its role in viral entry and its ability to elicit an immune response.
Antibody Research: Researchers study the interactions between GP120 and neutralizing antibodies to develop therapeutic antibodies that can block HIV infection.
Drug Development: The peptide is used in screening assays to identify potential inhibitors of HIV entry.
Structural Biology: Structural studies of the GP120 peptide provide insights into its conformation and interactions with host cell receptors, aiding in the design of antiviral drugs.
Mécanisme D'action
The HIV (GP120) antigenic peptide exerts its effects by binding to the CD4 receptor on host cells, followed by interaction with co-receptors CCR5 or CXCR4 . This binding induces conformational changes in the GP120 peptide, exposing the GP41 subunit, which facilitates the fusion of the viral and host cell membranes . The molecular targets involved in this process include the CD4 receptor, CCR5, and CXCR4 co-receptors .
Comparaison Avec Des Composés Similaires
Similar Compounds:
GP41: Another subunit of the HIV envelope glycoprotein complex, involved in membrane fusion.
VRC01: A broadly neutralizing antibody that targets the CD4 binding site on GP120.
NBD Analogues: Small molecules that bind to the GP120 cavity and inhibit HIV entry.
Uniqueness of GP120: The HIV (GP120) antigenic peptide is unique due to its critical role in the initial stages of HIV infection and its ability to elicit a strong immune response. Unlike other similar compounds, GP120 is directly involved in the binding and entry of the virus into host cells, making it a prime target for vaccine and therapeutic development .
Activité Biologique
The HIV (gp120) antigenic peptide plays a crucial role in the pathogenesis of HIV infection and serves as a significant target for therapeutic interventions. This article explores the biological activity of gp120-derived peptides, focusing on their mechanisms of action, efficacy in inhibiting HIV replication, and potential applications in vaccine development.
Overview of gp120
HIV-1 gp120 is a glycoprotein that forms part of the envelope of the virus and is essential for its entry into host cells. It interacts with the CD4 receptor on T lymphocytes and other immune cells, facilitating viral entry. The structure of gp120 includes several conserved and variable regions, which are critical for its function and interaction with host receptors.
Inhibition of HIV Replication
Research has demonstrated that certain synthetic peptides derived from the gp120 sequence can inhibit HIV-1 replication in vitro. For instance, two specific peptides were shown to block HIV-1 replication in macrophages and T lymphocytes through a sequence-dependent mechanism. These peptides also prevented the binding of anti-CXCR4 antibodies to CXCR4, thereby inhibiting intracellular calcium influx in response to chemokines like CXCL12/SDF-1α .
Interaction with CD4 and Coreceptors
The binding of gp120 to CD4 triggers conformational changes that expose binding sites for chemokine coreceptors (CCR5 or CXCR4). Peptides designed to mimic or block these interactions can effectively inhibit viral entry. For example, a peptide identified as G1 was found to inhibit the interaction between gp120 and CD4 with an IC50 value of approximately 50 µM, while its hexameric derivative showed an improved IC50 of 6 µM .
Peptide Nanofiber Vaccines
A study demonstrated that varying the density of gp120 on peptide nanofibers could enhance antibody responses against HIV-1. Increased antigen valency correlated with higher antibody binding and germinal center responses, showcasing the potential for peptide nanofiber platforms in vaccine development .
Structural Insights into Peptide Design
Structural analyses have revealed key residues involved in the interaction between gp120 and its receptors. For instance, peptides derived from broadly neutralizing antibodies (bNAbs) were designed based on co-crystallized structures with gp120. These peptides showed stable interactions with critical residues in the CD4 binding pocket, suggesting their potential as effective neutralizing agents .
Data Table: Summary of Key Peptides and Their Activities
Propriétés
Numéro CAS |
198636-94-1 |
---|---|
Formule moléculaire |
C117H211N41O31S |
Poids moléculaire |
2720.291 |
InChI |
InChI=1S/C117H211N41O31S/c1-14-63(10)90(155-102(177)68(29-15-19-45-118)140-83(161)56-136-93(168)67(122)58-190)109(184)149-77(41-44-86(165)166)112(187)158-54-28-37-80(158)104(179)151-79(55-59(2)3)94(169)137-57-84(162)152-87(60(4)5)106(181)139-65(12)111(186)157-53-27-38-81(157)105(180)156-91(66(13)159)110(185)147-69(30-16-20-46-119)95(170)138-64(11)92(167)141-70(31-17-21-47-120)96(171)143-72(33-23-49-132-114(124)125)97(172)145-74(35-25-51-134-116(128)129)103(178)153-89(62(8)9)108(183)154-88(61(6)7)107(182)148-75(39-42-82(123)160)100(175)144-73(34-24-50-133-115(126)127)98(173)146-76(40-43-85(163)164)101(176)142-71(32-18-22-48-121)99(174)150-78(113(188)189)36-26-52-135-117(130)131/h59-81,87-91,159,190H,14-58,118-122H2,1-13H3,(H2,123,160)(H,136,168)(H,137,169)(H,138,170)(H,139,181)(H,140,161)(H,141,167)(H,142,176)(H,143,171)(H,144,175)(H,145,172)(H,146,173)(H,147,185)(H,148,182)(H,149,184)(H,150,174)(H,151,179)(H,152,162)(H,153,178)(H,154,183)(H,155,177)(H,156,180)(H,163,164)(H,165,166)(H,188,189)(H4,124,125,132)(H4,126,127,133)(H4,128,129,134)(H4,130,131,135)/t63-,64-,65-,66+,67-,68-,69-,70-,71-,72-,73-,74-,75-,76-,77-,78-,79-,80-,81-,87-,88-,89-,90-,91-/m0/s1 |
Clé InChI |
CBJINRXLSYHNOL-QEZKGTQVSA-N |
SMILES |
CCC(C)C(C(=O)NC(CCC(=O)O)C(=O)N1CCCC1C(=O)NC(CC(C)C)C(=O)NCC(=O)NC(C(C)C)C(=O)NC(C)C(=O)N2CCCC2C(=O)NC(C(C)O)C(=O)NC(CCCCN)C(=O)NC(C)C(=O)NC(CCCCN)C(=O)NC(CCCNC(=N)N)C(=O)NC(CCCNC(=N)N)C(=O)NC(C(C)C)C(=O)NC(C(C)C)C(=O)NC(CCC(=O)N)C(=O)NC(CCCNC(=N)N)C(=O)NC(CCC(=O)O)C(=O)NC(CCCCN)C(=O)NC(CCCNC(=N)N)C(=O)O)NC(=O)C(CCCCN)NC(=O)CNC(=O)C(CS)N |
Origine du produit |
United States |
Avertissement et informations sur les produits de recherche in vitro
Veuillez noter que tous les articles et informations sur les produits présentés sur BenchChem sont destinés uniquement à des fins informatives. Les produits disponibles à l'achat sur BenchChem sont spécifiquement conçus pour des études in vitro, qui sont réalisées en dehors des organismes vivants. Les études in vitro, dérivées du terme latin "in verre", impliquent des expériences réalisées dans des environnements de laboratoire contrôlés à l'aide de cellules ou de tissus. Il est important de noter que ces produits ne sont pas classés comme médicaments et n'ont pas reçu l'approbation de la FDA pour la prévention, le traitement ou la guérison de toute condition médicale, affection ou maladie. Nous devons souligner que toute forme d'introduction corporelle de ces produits chez les humains ou les animaux est strictement interdite par la loi. Il est essentiel de respecter ces directives pour assurer la conformité aux normes légales et éthiques en matière de recherche et d'expérimentation.