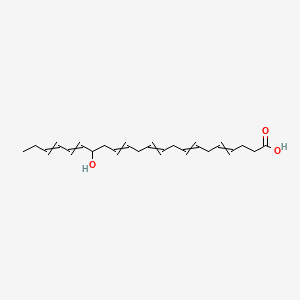
16-Hydroxydocosa-4,7,10,13,17,19-hexaenoic acid
Vue d'ensemble
Description
16-Hydroxydocosa-4,7,10,13,17,19-hexaenoic acid is a polyunsaturated fatty acid . It is an autoxidation product of docosahexaenoic acid (DHA) in vitro.
Molecular Structure Analysis
The molecular formula of 16-Hydroxydocosa-4,7,10,13,17,19-hexaenoic acid is C22H32O3 . It consists of 4 Z,7 Z,10 Z,13 Z,17 E,19 Z -docosahexaenoic acid bearing an additional 16-hydroxy substituent .Physical And Chemical Properties Analysis
The molecular weight of 16-Hydroxydocosa-4,7,10,13,17,19-hexaenoic acid is 344.495 . The net charge is 0 .Applications De Recherche Scientifique
Chemical Structure and Properties
“16-Hydroxydocosa-4,7,10,13,17,19-hexaenoic acid” is a hydroxydocosahexaenoic acid that consists of 4Z,7Z,10Z,13Z,17E,19Z-docosahexaenoic acid bearing an additional 16-hydroxy substituent . It has a molecular formula of C22H32O3 and an average mass of 344.48770 .
Biological Role
This compound plays a role as a metabolite, which is any intermediate or product resulting from metabolism . Metabolites play crucial roles in various biological processes, including energy production, growth, development, and reproduction.
Anti-Inflammatory Role
It has been shown to play a major anti-inflammatory role . For instance, it can prevent lipopolysaccharide (LPS)-induced dendritic cell maturation, which is characterized by a lack of proinflammatory cytokine production .
Propriétés
IUPAC Name |
16-hydroxydocosa-4,7,10,13,17,19-hexaenoic acid | |
---|---|---|
Source | PubChem | |
URL | https://pubchem.ncbi.nlm.nih.gov | |
Description | Data deposited in or computed by PubChem | |
InChI |
InChI=1S/C22H32O3/c1-2-3-4-15-18-21(23)19-16-13-11-9-7-5-6-8-10-12-14-17-20-22(24)25/h3-4,6-9,12-16,18,21,23H,2,5,10-11,17,19-20H2,1H3,(H,24,25) | |
Source | PubChem | |
URL | https://pubchem.ncbi.nlm.nih.gov | |
Description | Data deposited in or computed by PubChem | |
InChI Key |
CSXQXWHAGLIFIH-UHFFFAOYSA-N | |
Source | PubChem | |
URL | https://pubchem.ncbi.nlm.nih.gov | |
Description | Data deposited in or computed by PubChem | |
Canonical SMILES |
CCC=CC=CC(CC=CCC=CCC=CCC=CCCC(=O)O)O | |
Source | PubChem | |
URL | https://pubchem.ncbi.nlm.nih.gov | |
Description | Data deposited in or computed by PubChem | |
Molecular Formula |
C22H32O3 | |
Source | PubChem | |
URL | https://pubchem.ncbi.nlm.nih.gov | |
Description | Data deposited in or computed by PubChem | |
DSSTOX Substance ID |
DTXSID40694008 | |
Record name | 16-Hydroxydocosa-4,7,10,13,17,19-hexaenoic acid | |
Source | EPA DSSTox | |
URL | https://comptox.epa.gov/dashboard/DTXSID40694008 | |
Description | DSSTox provides a high quality public chemistry resource for supporting improved predictive toxicology. | |
Molecular Weight |
344.5 g/mol | |
Source | PubChem | |
URL | https://pubchem.ncbi.nlm.nih.gov | |
Description | Data deposited in or computed by PubChem | |
Product Name |
16-Hydroxydocosa-4,7,10,13,17,19-hexaenoic acid | |
CAS RN |
90780-51-1 | |
Record name | 16-Hydroxydocosa-4,7,10,13,17,19-hexaenoic acid | |
Source | EPA DSSTox | |
URL | https://comptox.epa.gov/dashboard/DTXSID40694008 | |
Description | DSSTox provides a high quality public chemistry resource for supporting improved predictive toxicology. | |
Retrosynthesis Analysis
AI-Powered Synthesis Planning: Our tool employs the Template_relevance Pistachio, Template_relevance Bkms_metabolic, Template_relevance Pistachio_ringbreaker, Template_relevance Reaxys, Template_relevance Reaxys_biocatalysis model, leveraging a vast database of chemical reactions to predict feasible synthetic routes.
One-Step Synthesis Focus: Specifically designed for one-step synthesis, it provides concise and direct routes for your target compounds, streamlining the synthesis process.
Accurate Predictions: Utilizing the extensive PISTACHIO, BKMS_METABOLIC, PISTACHIO_RINGBREAKER, REAXYS, REAXYS_BIOCATALYSIS database, our tool offers high-accuracy predictions, reflecting the latest in chemical research and data.
Strategy Settings
Precursor scoring | Relevance Heuristic |
---|---|
Min. plausibility | 0.01 |
Model | Template_relevance |
Template Set | Pistachio/Bkms_metabolic/Pistachio_ringbreaker/Reaxys/Reaxys_biocatalysis |
Top-N result to add to graph | 6 |
Feasible Synthetic Routes
Avertissement et informations sur les produits de recherche in vitro
Veuillez noter que tous les articles et informations sur les produits présentés sur BenchChem sont destinés uniquement à des fins informatives. Les produits disponibles à l'achat sur BenchChem sont spécifiquement conçus pour des études in vitro, qui sont réalisées en dehors des organismes vivants. Les études in vitro, dérivées du terme latin "in verre", impliquent des expériences réalisées dans des environnements de laboratoire contrôlés à l'aide de cellules ou de tissus. Il est important de noter que ces produits ne sont pas classés comme médicaments et n'ont pas reçu l'approbation de la FDA pour la prévention, le traitement ou la guérison de toute condition médicale, affection ou maladie. Nous devons souligner que toute forme d'introduction corporelle de ces produits chez les humains ou les animaux est strictement interdite par la loi. Il est essentiel de respecter ces directives pour assurer la conformité aux normes légales et éthiques en matière de recherche et d'expérimentation.