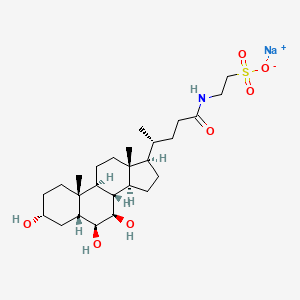
Acide tauro-bêta-muricholique (sel de sodium)
- Cliquez sur DEMANDE RAPIDE pour recevoir un devis de notre équipe d'experts.
- Avec des produits de qualité à un prix COMPÉTITIF, vous pouvez vous concentrer davantage sur votre recherche.
Vue d'ensemble
Description
Tauro-β-muricholic acid (TβMCA) is a competitive and reversible antagonist of the farnesoid X receptor (FXR; IC50 = 40 µM) and a taurine-conjugated form of the murine-specific primary bile acid β-muricholic acid. TβMCA accumulates in germ-free mice under normal conditions but is reduced after colonization with feces from a human donor. TβMCA is increased in the intestines of mice resistant to high-fat diet-induced obesity, fatty liver, and diabetes.
Tauro-β-muricholic acid (TβMCA) is a potent competitive and reversible antagonist of the farnesoid X receptor (FXR) (IC50 = 40 µM) that binds bile acids. TβMCA accumulates in germ-free mice under normal conditions but is reduced after colonization with feces from a human donor. TβMCA is increased in the intestines of mice resistant to high-fat diet-induced obesity, fatty liver, and diabetes.
Applications De Recherche Scientifique
Tauro-beta-muricholic Acid (sodium salt) has a wide range of scientific research applications:
Chemistry: Used as a reagent in organic synthesis and as a standard in analytical chemistry.
Biology: Studied for its role in bile acid metabolism and its effects on gut microbiota.
Medicine: Investigated for its potential therapeutic effects in metabolic diseases such as obesity, diabetes, and fatty liver disease.
Industry: Used in the development of pharmaceuticals and as a biochemical reagent
Mécanisme D'action
- Tauro-beta-muricholic Acid (TβMCA) primarily interacts with two key targets:
- Bile Acid Receptor (FXR) : TβMCA acts as a competitive and reversible antagonist of the farnesoid X receptor (FXR). FXR is a nuclear receptor involved in regulating bile acid homeostasis, lipid metabolism, and glucose control .
Target of Action
Analyse Biochimique
Biochemical Properties
Tauro-beta-muricholic Acid (sodium salt) plays a significant role in biochemical reactions. It interacts with the farnesoid X receptor (FXR), a nuclear receptor that regulates the synthesis and transport of bile acids . By acting as a competitive and reversible antagonist of FXR, Tauro-beta-muricholic Acid (sodium salt) can influence the levels of bile acids in the body .
Cellular Effects
Tauro-beta-muricholic Acid (sodium salt) has notable effects on various types of cells and cellular processes. It influences cell function by interacting with the FXR, which plays a crucial role in regulating bile acid levels, lipid metabolism, and glucose homeostasis . This interaction can impact cell signaling pathways, gene expression, and cellular metabolism .
Molecular Mechanism
The molecular mechanism of Tauro-beta-muricholic Acid (sodium salt) involves its interaction with the FXR. As a competitive and reversible antagonist, it binds to the FXR, preventing the receptor from carrying out its normal function of regulating bile acid synthesis . This interaction can lead to changes in gene expression and enzyme activity .
Temporal Effects in Laboratory Settings
In laboratory settings, the effects of Tauro-beta-muricholic Acid (sodium salt) can change over time. It has been observed to accumulate in germ-free mice under normal conditions but is reduced after colonization with feces from a human donor . This suggests that the compound’s stability and degradation may be influenced by the presence of certain gut microbiota .
Dosage Effects in Animal Models
The effects of Tauro-beta-muricholic Acid (sodium salt) can vary with different dosages in animal models. While specific dosage effects have not been detailed in the search results, it is known that the compound accumulates in germ-free mice under normal conditions .
Metabolic Pathways
Tauro-beta-muricholic Acid (sodium salt) is involved in the metabolic pathway of bile acid synthesis. It interacts with the FXR, a key regulator of this pathway . The compound’s antagonistic action on the FXR can influence the levels of bile acids in the body .
Transport and Distribution
The transport and distribution of Tauro-beta-muricholic Acid (sodium salt) within cells and tissues are likely influenced by its interaction with the FXR . Specific details about its transporters or binding proteins were not found in the search results.
Subcellular Localization
Given its role as an antagonist of the FXR, it is likely to be found in locations where this receptor is present, such as the nucleus .
Méthodes De Préparation
Synthetic Routes and Reaction Conditions
The synthesis of Tauro-beta-muricholic Acid (sodium salt) involves the conjugation of beta-muricholic acid with taurine. The reaction typically requires the activation of the carboxyl group of beta-muricholic acid, followed by its reaction with taurine in the presence of coupling agents such as N,N’-dicyclohexylcarbodiimide (DCC) and 4-dimethylaminopyridine (DMAP). The reaction is carried out under mild conditions to prevent degradation of the product .
Industrial Production Methods
Industrial production of Tauro-beta-muricholic Acid (sodium salt) follows similar synthetic routes but on a larger scale. The process involves the use of high-purity reagents and controlled reaction conditions to ensure the consistency and quality of the final product. The compound is typically purified using chromatographic techniques and crystallized to obtain a high-purity product .
Analyse Des Réactions Chimiques
Types of Reactions
Tauro-beta-muricholic Acid (sodium salt) undergoes various chemical reactions, including:
Oxidation: The hydroxyl groups in the compound can be oxidized to form ketones or aldehydes.
Reduction: The compound can be reduced to form alcohols.
Substitution: The sulfonic acid group can undergo substitution reactions with various nucleophiles.
Common Reagents and Conditions
Oxidation: Common oxidizing agents include potassium permanganate (KMnO4) and chromium trioxide (CrO3).
Reduction: Reducing agents such as lithium aluminum hydride (LiAlH4) and sodium borohydride (NaBH4) are used.
Substitution: Nucleophiles such as amines and thiols can react with the sulfonic acid group under basic conditions.
Major Products Formed
Oxidation: Formation of ketones or aldehydes.
Reduction: Formation of alcohols.
Substitution: Formation of sulfonamide or sulfonate derivatives.
Comparaison Avec Des Composés Similaires
Similar Compounds
- Tauro-alpha-muricholic Acid (sodium salt)
- Tauro-omega-muricholic Acid (sodium salt)
- Beta-muricholic Acid
Comparison
Tauro-beta-muricholic Acid (sodium salt) is unique in its specific antagonistic action on FXR, which distinguishes it from other similar compounds. While Tauro-alpha-muricholic Acid and Tauro-omega-muricholic Acid also interact with bile acid receptors, their effects and potency differ. Beta-muricholic Acid, being the unconjugated form, has different solubility and bioavailability properties .
Propriétés
IUPAC Name |
sodium;2-[[(4R)-4-[(3R,5R,6S,7R,8S,9S,10R,13R,14S,17R)-3,6,7-trihydroxy-10,13-dimethyl-2,3,4,5,6,7,8,9,11,12,14,15,16,17-tetradecahydro-1H-cyclopenta[a]phenanthren-17-yl]pentanoyl]amino]ethanesulfonate |
Source
|
---|---|---|
Source | PubChem | |
URL | https://pubchem.ncbi.nlm.nih.gov | |
Description | Data deposited in or computed by PubChem | |
InChI |
InChI=1S/C26H45NO7S.Na/c1-15(4-7-21(29)27-12-13-35(32,33)34)17-5-6-18-22-19(9-11-25(17,18)2)26(3)10-8-16(28)14-20(26)23(30)24(22)31;/h15-20,22-24,28,30-31H,4-14H2,1-3H3,(H,27,29)(H,32,33,34);/q;+1/p-1/t15-,16-,17-,18+,19+,20+,22+,23+,24-,25-,26-;/m1./s1 |
Source
|
Source | PubChem | |
URL | https://pubchem.ncbi.nlm.nih.gov | |
Description | Data deposited in or computed by PubChem | |
InChI Key |
NYXROOLWUZIWRB-GPHZYVDLSA-M |
Source
|
Source | PubChem | |
URL | https://pubchem.ncbi.nlm.nih.gov | |
Description | Data deposited in or computed by PubChem | |
Canonical SMILES |
CC(CCC(=O)NCCS(=O)(=O)[O-])C1CCC2C1(CCC3C2C(C(C4C3(CCC(C4)O)C)O)O)C.[Na+] |
Source
|
Source | PubChem | |
URL | https://pubchem.ncbi.nlm.nih.gov | |
Description | Data deposited in or computed by PubChem | |
Isomeric SMILES |
C[C@H](CCC(=O)NCCS(=O)(=O)[O-])[C@H]1CC[C@@H]2[C@@]1(CC[C@H]3[C@H]2[C@H]([C@H]([C@H]4[C@@]3(CC[C@H](C4)O)C)O)O)C.[Na+] |
Source
|
Source | PubChem | |
URL | https://pubchem.ncbi.nlm.nih.gov | |
Description | Data deposited in or computed by PubChem | |
Molecular Formula |
C26H44NNaO7S |
Source
|
Source | PubChem | |
URL | https://pubchem.ncbi.nlm.nih.gov | |
Description | Data deposited in or computed by PubChem | |
Molecular Weight |
537.7 g/mol |
Source
|
Source | PubChem | |
URL | https://pubchem.ncbi.nlm.nih.gov | |
Description | Data deposited in or computed by PubChem | |
Avertissement et informations sur les produits de recherche in vitro
Veuillez noter que tous les articles et informations sur les produits présentés sur BenchChem sont destinés uniquement à des fins informatives. Les produits disponibles à l'achat sur BenchChem sont spécifiquement conçus pour des études in vitro, qui sont réalisées en dehors des organismes vivants. Les études in vitro, dérivées du terme latin "in verre", impliquent des expériences réalisées dans des environnements de laboratoire contrôlés à l'aide de cellules ou de tissus. Il est important de noter que ces produits ne sont pas classés comme médicaments et n'ont pas reçu l'approbation de la FDA pour la prévention, le traitement ou la guérison de toute condition médicale, affection ou maladie. Nous devons souligner que toute forme d'introduction corporelle de ces produits chez les humains ou les animaux est strictement interdite par la loi. Il est essentiel de respecter ces directives pour assurer la conformité aux normes légales et éthiques en matière de recherche et d'expérimentation.