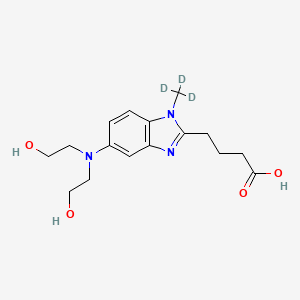
Dihydroxy Bendamustine-d3
- Cliquez sur DEMANDE RAPIDE pour recevoir un devis de notre équipe d'experts.
- Avec des produits de qualité à un prix COMPÉTITIF, vous pouvez vous concentrer davantage sur votre recherche.
Vue d'ensemble
Description
Dihydroxy Bendamustine-d3 is a derivative of Bendamustine, a bifunctional alkylating agent used primarily in the treatment of hematologic malignancies such as chronic lymphocytic leukemia and non-Hodgkin’s lymphoma . This compound is characterized by the presence of two hydroxyl groups, which are introduced through hydrolysis .
Méthodes De Préparation
The synthesis of Dihydroxy Bendamustine-d3 involves several steps, starting from Bendamustine hydrochloride. The process typically includes hydrolysis to introduce the hydroxyl groups. The synthetic route involves the following steps :
Hydrolysis: Bendamustine hydrochloride is subjected to hydrolysis to form monohydroxy and dihydroxy derivatives.
Protection/Deprotection: Benzyl-protection and deprotection of the amine and carboxylic acid groups are performed to ensure selective reactions.
Saponification: The ester groups are hydrolyzed to form the corresponding acids.
Ring-Opening Reaction: Oxirane rings are opened to introduce additional functional groups.
Esterification: Fischer or Steglish esterification is used to form ester linkages.
Analyse Des Réactions Chimiques
Dihydroxy Bendamustine-d3 undergoes several types of chemical reactions, including:
Oxidation: The hydroxyl groups can be oxidized to form ketones or aldehydes.
Reduction: The compound can be reduced to form alcohols or amines.
Substitution: The hydroxyl groups can be substituted with other functional groups such as halides or amines.
Common reagents used in these reactions include oxidizing agents like potassium permanganate, reducing agents like lithium aluminum hydride, and halogenating agents like thionyl chloride . Major products formed from these reactions include various derivatives with modified functional groups.
Applications De Recherche Scientifique
Dihydroxy Bendamustine-d3 has several scientific research applications, including:
Chemistry: Used as a model compound to study the reactivity of hydroxylated alkylating agents.
Biology: Investigated for its effects on cellular processes and DNA interactions.
Medicine: Explored for its potential use in targeted cancer therapies due to its ability to form crosslinks with DNA.
Industry: Utilized in the development of new pharmaceuticals and chemical intermediates
Mécanisme D'action
The mechanism of action of Dihydroxy Bendamustine-d3 involves the formation of electrophilic alkyl groups that covalently bond to DNA molecules. This results in intra- and inter-strand crosslinks, leading to DNA damage and cell death . The compound can induce cell death through both apoptotic and non-apoptotic pathways, making it effective even in cells lacking functional apoptotic pathways .
Comparaison Avec Des Composés Similaires
Dihydroxy Bendamustine-d3 is unique compared to other similar compounds due to its dual hydroxyl groups, which enhance its reactivity and potential applications. Similar compounds include:
Monohydroxy Bendamustine: A derivative with a single hydroxyl group, less reactive than this compound.
N-desmethyl Bendamustine: A metabolite with a demethylated nitrogen atom, differing in its metabolic pathway and activity
Propriétés
IUPAC Name |
4-[5-[bis(2-hydroxyethyl)amino]-1-(trideuteriomethyl)benzimidazol-2-yl]butanoic acid |
Source
|
---|---|---|
Source | PubChem | |
URL | https://pubchem.ncbi.nlm.nih.gov | |
Description | Data deposited in or computed by PubChem | |
InChI |
InChI=1S/C16H23N3O4/c1-18-14-6-5-12(19(7-9-20)8-10-21)11-13(14)17-15(18)3-2-4-16(22)23/h5-6,11,20-21H,2-4,7-10H2,1H3,(H,22,23)/i1D3 |
Source
|
Source | PubChem | |
URL | https://pubchem.ncbi.nlm.nih.gov | |
Description | Data deposited in or computed by PubChem | |
InChI Key |
XQMDIDKYVZPCNV-FIBGUPNXSA-N |
Source
|
Source | PubChem | |
URL | https://pubchem.ncbi.nlm.nih.gov | |
Description | Data deposited in or computed by PubChem | |
Canonical SMILES |
CN1C2=C(C=C(C=C2)N(CCO)CCO)N=C1CCCC(=O)O |
Source
|
Source | PubChem | |
URL | https://pubchem.ncbi.nlm.nih.gov | |
Description | Data deposited in or computed by PubChem | |
Isomeric SMILES |
[2H]C([2H])([2H])N1C2=C(C=C(C=C2)N(CCO)CCO)N=C1CCCC(=O)O |
Source
|
Source | PubChem | |
URL | https://pubchem.ncbi.nlm.nih.gov | |
Description | Data deposited in or computed by PubChem | |
Molecular Formula |
C16H23N3O4 |
Source
|
Source | PubChem | |
URL | https://pubchem.ncbi.nlm.nih.gov | |
Description | Data deposited in or computed by PubChem | |
Molecular Weight |
324.39 g/mol |
Source
|
Source | PubChem | |
URL | https://pubchem.ncbi.nlm.nih.gov | |
Description | Data deposited in or computed by PubChem | |
Avertissement et informations sur les produits de recherche in vitro
Veuillez noter que tous les articles et informations sur les produits présentés sur BenchChem sont destinés uniquement à des fins informatives. Les produits disponibles à l'achat sur BenchChem sont spécifiquement conçus pour des études in vitro, qui sont réalisées en dehors des organismes vivants. Les études in vitro, dérivées du terme latin "in verre", impliquent des expériences réalisées dans des environnements de laboratoire contrôlés à l'aide de cellules ou de tissus. Il est important de noter que ces produits ne sont pas classés comme médicaments et n'ont pas reçu l'approbation de la FDA pour la prévention, le traitement ou la guérison de toute condition médicale, affection ou maladie. Nous devons souligner que toute forme d'introduction corporelle de ces produits chez les humains ou les animaux est strictement interdite par la loi. Il est essentiel de respecter ces directives pour assurer la conformité aux normes légales et éthiques en matière de recherche et d'expérimentation.