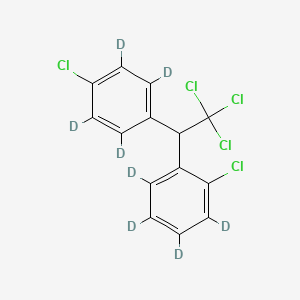
2,4'-DDT-d8
Vue d'ensemble
Description
1-Chloro-2,3,4,5-tetradeuterio-6-[2,2,2-trichloro-1-(4-chloro-2,3,5,6-tetradeuteriophenyl)ethyl]benzene, also known as 1-Chloro-2,3,4,5-tetradeuterio-6-[2,2,2-trichloro-1-(4-chloro-2,3,5,6-tetradeuteriophenyl)ethyl]benzene, is a useful research compound. Its molecular formula is C14H9Cl5 and its molecular weight is 362.525. The purity is usually 95%.
BenchChem offers high-quality 1-Chloro-2,3,4,5-tetradeuterio-6-[2,2,2-trichloro-1-(4-chloro-2,3,5,6-tetradeuteriophenyl)ethyl]benzene suitable for many research applications. Different packaging options are available to accommodate customers' requirements. Please inquire for more information about 1-Chloro-2,3,4,5-tetradeuterio-6-[2,2,2-trichloro-1-(4-chloro-2,3,5,6-tetradeuteriophenyl)ethyl]benzene including the price, delivery time, and more detailed information at info@benchchem.com.
Applications De Recherche Scientifique
Composés marqués par des isotopes stables
2,4'-DDT-d8 est un composé marqué par un isotope stable . Ces types de composés sont utilisés dans divers domaines de recherche scientifique, notamment la biochimie, la pharmacologie et les sciences de l'environnement. Ils sont particulièrement utiles dans les études de traçage isotopique, où ils peuvent aider à suivre le flux des atomes dans les voies métaboliques.
Étalons de référence pour les pesticides
Ce composé est utilisé comme étalon de référence dans la recherche sur les pesticides . Les étalons de référence sont essentiels en chimie analytique car ils garantissent la précision de la méthode analytique. Ils sont utilisés pour calibrer l'équipement et valider les résultats.
Tests alimentaires et des boissons
this compound est utilisé pour les tests analytiques dans le secteur alimentaire et des boissons . Il peut être utilisé pour tester la présence de pesticides dans les produits alimentaires et les boissons, garantissant ainsi leur sécurité pour la consommation.
Analyse environnementale
Ce composé est utilisé dans l'analyse environnementale . Il peut être utilisé pour tester la présence de pesticides dans divers échantillons environnementaux, tels que le sol ou l'eau. Cela permet de surveiller l'impact environnemental de l'utilisation des pesticides.
Étalon de substitution de récupération
4,4'-DDT-d8 peut être utilisé comme étalon de substitution de récupération pour la quantification de 4,4'-DDT dans l'eau interstitielle marine . Cela implique l'utilisation d'une microextraction en phase solide à l'équilibre (SPME) suivie d'une désorption thermique automatisée couplée à la chromatographie en phase gazeuse/spectrométrie de masse (TD-GC-MS) utilisant des fibres optiques jetables.
Évaluations environnementales du dicofol
Le composé est utilisé dans l'analyse du dicofol, un pesticide bien connu . Il aide à la quantification et à la détection du dicofol dans les échantillons environnementaux, contribuant ainsi à la compréhension de la présence et de l'impact du dicofol dans l'environnement.
Mécanisme D'action
Target of Action
It’s known that ddt and its derivatives generally target the nervous system of insects, interfering with the sodium channels in their neurons .
Mode of Action
Ddt and its derivatives are known to interfere with the normal functioning of the nervous system in insects by opening sodium channels in their neurons, leading to nerve impulse disruption .
Biochemical Pathways
Ddt and its derivatives are known to affect various biochemical pathways, particularly those involved in nerve signal transmission .
Pharmacokinetics
They are also resistant to metabolism, leading to their persistence in the environment and in organisms .
Result of Action
Ddt and its derivatives are known to cause nerve impulse disruption in insects, leading to their paralysis and eventual death .
Action Environment
Environmental factors can influence the action, efficacy, and stability of 2,4’-DDT-d8 . Factors such as temperature, soil organic matter, and other environmental conditions can affect the degradation and distribution of DDT and its derivatives in the environment .
Analyse Biochimique
Biochemical Properties
The biochemical properties of 2,4’-DDT-d8 are largely determined by its structural similarity to 2,4’-DDT. It is expected to interact with similar enzymes, proteins, and other biomolecules as 2,4’-DDT. The presence of deuterium atoms can potentially affect these interactions due to the kinetic isotope effect
Cellular Effects
The cellular effects of 2,4’-DDT-d8 are likely to be similar to those of 2,4’-DDT, given their structural similarity. 2,4’-DDT has been shown to have various effects on cells, including alterations in cell signaling pathways, gene expression, and cellular metabolism
Molecular Mechanism
The molecular mechanism of action of 2,4’-DDT-d8 is not well-understood. Given its structural similarity to 2,4’-DDT, it is likely that 2,4’-DDT-d8 exerts its effects at the molecular level through similar mechanisms. These may include binding interactions with biomolecules, enzyme inhibition or activation, and changes in gene expression
Temporal Effects in Laboratory Settings
The temporal effects of 2,4’-DDT-d8 in laboratory settings, including its stability, degradation, and long-term effects on cellular function, are not well-documented. Given its structural similarity to 2,4’-DDT, it is likely that 2,4’-DDT-d8 exhibits similar temporal trends. The presence of deuterium atoms may influence these trends due to the kinetic isotope effect .
Dosage Effects in Animal Models
The effects of 2,4’-DDT-d8 at different dosages in animal models are not well-studied. It is likely that the effects of 2,4’-DDT-d8 vary with dosage, similar to 2,4’-DDT. Studies have shown that 2,4’-DDT can have toxic or adverse effects at high doses
Metabolic Pathways
The metabolic pathways involving 2,4’-DDT-d8 are not well-understood. Given its structural similarity to 2,4’-DDT, it is likely that 2,4’-DDT-d8 is involved in similar metabolic pathways. These may involve interactions with various enzymes or cofactors, and could potentially affect metabolic flux or metabolite levels
Transport and Distribution
The transport and distribution of 2,4’-DDT-d8 within cells and tissues are not well-documented. Given its structural similarity to 2,4’-DDT, it is likely that 2,4’-DDT-d8 is transported and distributed in a similar manner. This could involve interactions with various transporters or binding proteins, and could potentially affect its localization or accumulation .
Activité Biologique
1-Chloro-2,3,4,5-tetradeuterio-6-[2,2,2-trichloro-1-(4-chloro-2,3,5,6-tetradeuteriophenyl)ethyl]benzene is a complex halogenated organic compound with potential applications in various fields including medicinal chemistry and environmental science. Understanding its biological activity is crucial for assessing its safety and efficacy in potential applications.
Chemical Structure
The compound features a chlorinated benzene ring with multiple deuterium substitutions and a trichlorinated side chain. This structure can influence its reactivity and interaction with biological systems.
Biological Activity Overview
Research on halogenated compounds has shown that they can exhibit a range of biological activities including antimicrobial, anti-inflammatory, and anticancer properties. The specific biological activities of 1-Chloro-2,3,4,5-tetradeuterio-6-[2,2,2-trichloro-1-(4-chloro-2,3,5,6-tetradeuteriophenyl)ethyl]benzene are still under investigation.
Antimicrobial Activity
Halogenated compounds often demonstrate antimicrobial properties. For instance:
- Study Findings : A study indicated that chlorinated phenolic compounds exhibited significant antibacterial activity against various strains of bacteria. The presence of multiple chlorine atoms can enhance this activity by increasing membrane permeability in bacterial cells .
Cytotoxicity and Anticancer Activity
The cytotoxic effects of chlorinated compounds have been documented in several studies:
- Case Study : In vitro studies have shown that chlorinated benzene derivatives can induce apoptosis in cancer cell lines. The mechanism often involves the generation of reactive oxygen species (ROS) leading to oxidative stress and subsequent cell death .
Data Table: Summary of Biological Activities
Detailed Research Findings
- Antimicrobial Studies :
- Cytotoxic Mechanisms :
- In Vivo Studies :
Propriétés
IUPAC Name |
1-chloro-2,3,4,5-tetradeuterio-6-[2,2,2-trichloro-1-(4-chloro-2,3,5,6-tetradeuteriophenyl)ethyl]benzene | |
---|---|---|
Source | PubChem | |
URL | https://pubchem.ncbi.nlm.nih.gov | |
Description | Data deposited in or computed by PubChem | |
InChI |
InChI=1S/C14H9Cl5/c15-10-7-5-9(6-8-10)13(14(17,18)19)11-3-1-2-4-12(11)16/h1-8,13H/i1D,2D,3D,4D,5D,6D,7D,8D | |
Source | PubChem | |
URL | https://pubchem.ncbi.nlm.nih.gov | |
Description | Data deposited in or computed by PubChem | |
InChI Key |
CVUGPAFCQJIYDT-PGRXLJNUSA-N | |
Source | PubChem | |
URL | https://pubchem.ncbi.nlm.nih.gov | |
Description | Data deposited in or computed by PubChem | |
Canonical SMILES |
C1=CC=C(C(=C1)C(C2=CC=C(C=C2)Cl)C(Cl)(Cl)Cl)Cl | |
Source | PubChem | |
URL | https://pubchem.ncbi.nlm.nih.gov | |
Description | Data deposited in or computed by PubChem | |
Isomeric SMILES |
[2H]C1=C(C(=C(C(=C1[2H])C(C2=C(C(=C(C(=C2[2H])[2H])Cl)[2H])[2H])C(Cl)(Cl)Cl)Cl)[2H])[2H] | |
Source | PubChem | |
URL | https://pubchem.ncbi.nlm.nih.gov | |
Description | Data deposited in or computed by PubChem | |
Molecular Formula |
C14H9Cl5 | |
Source | PubChem | |
URL | https://pubchem.ncbi.nlm.nih.gov | |
Description | Data deposited in or computed by PubChem | |
DSSTOX Substance ID |
DTXSID501129305 | |
Record name | Benzene-1,2,3,4-d4, 5-chloro-6-[2,2,2-trichloro-1-(4-chlorophenyl-2,3,5,6-d4)ethyl]- | |
Source | EPA DSSTox | |
URL | https://comptox.epa.gov/dashboard/DTXSID501129305 | |
Description | DSSTox provides a high quality public chemistry resource for supporting improved predictive toxicology. | |
Molecular Weight |
362.5 g/mol | |
Source | PubChem | |
URL | https://pubchem.ncbi.nlm.nih.gov | |
Description | Data deposited in or computed by PubChem | |
CAS No. |
221899-88-3 | |
Record name | Benzene-1,2,3,4-d4, 5-chloro-6-[2,2,2-trichloro-1-(4-chlorophenyl-2,3,5,6-d4)ethyl]- | |
Source | CAS Common Chemistry | |
URL | https://commonchemistry.cas.org/detail?cas_rn=221899-88-3 | |
Description | CAS Common Chemistry is an open community resource for accessing chemical information. Nearly 500,000 chemical substances from CAS REGISTRY cover areas of community interest, including common and frequently regulated chemicals, and those relevant to high school and undergraduate chemistry classes. This chemical information, curated by our expert scientists, is provided in alignment with our mission as a division of the American Chemical Society. | |
Explanation | The data from CAS Common Chemistry is provided under a CC-BY-NC 4.0 license, unless otherwise stated. | |
Record name | Benzene-1,2,3,4-d4, 5-chloro-6-[2,2,2-trichloro-1-(4-chlorophenyl-2,3,5,6-d4)ethyl]- | |
Source | EPA DSSTox | |
URL | https://comptox.epa.gov/dashboard/DTXSID501129305 | |
Description | DSSTox provides a high quality public chemistry resource for supporting improved predictive toxicology. | |
Avertissement et informations sur les produits de recherche in vitro
Veuillez noter que tous les articles et informations sur les produits présentés sur BenchChem sont destinés uniquement à des fins informatives. Les produits disponibles à l'achat sur BenchChem sont spécifiquement conçus pour des études in vitro, qui sont réalisées en dehors des organismes vivants. Les études in vitro, dérivées du terme latin "in verre", impliquent des expériences réalisées dans des environnements de laboratoire contrôlés à l'aide de cellules ou de tissus. Il est important de noter que ces produits ne sont pas classés comme médicaments et n'ont pas reçu l'approbation de la FDA pour la prévention, le traitement ou la guérison de toute condition médicale, affection ou maladie. Nous devons souligner que toute forme d'introduction corporelle de ces produits chez les humains ou les animaux est strictement interdite par la loi. Il est essentiel de respecter ces directives pour assurer la conformité aux normes légales et éthiques en matière de recherche et d'expérimentation.