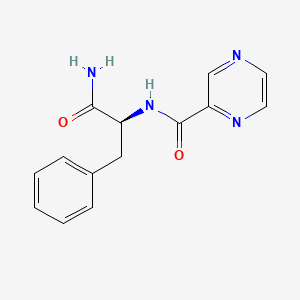
(S)-N-(1-Amino-1-oxo-3-phenylpropan-2-yl)pyrazine-2-carboxamide
Vue d'ensemble
Description
“(S)-N-(1-Amino-1-oxo-3-phenylpropan-2-yl)pyrazine-2-carboxamide” is a chemical compound with the molecular formula C14H14N4O2 . It is also known by other names such as Bortezomib (m3), N-[(1S)-2-Amino-2-oxo-1-(phenylmethyl)ethyl]-2-pyrazinecarboxamide, and has the CAS number 289472-80-6 .
Molecular Structure Analysis
The molecular structure of this compound includes a pyrazine ring, which is a six-membered aromatic ring with two nitrogen atoms, and an amide functional group attached to a phenyl group . The exact three-dimensional structure can be determined using techniques such as X-ray crystallography or NMR spectroscopy.Physical And Chemical Properties Analysis
This compound has a molecular weight of 270.29 g/mol . It has a predicted boiling point of 619.4°C and a predicted density of 1.280 g/cm3 . It is slightly soluble in chloroform, DMSO, ethanol, and methanol .Applications De Recherche Scientifique
Treatment of Pulmonary Fibrosis
Bortezomib has been shown to alleviate tissue fibrotic processes in a model of bleomycin-induced pulmonary fibrosis . The administration of bortezomib reduced the ratio of M2-polarized macrophages and decreased the accumulation of CD4 T cells expressing CXCR6 . This suggests that Bortezomib could be repurposed for the treatment of pulmonary fibrosis .
Multiple Myeloma Therapy
Bortezomib-based regimens are widely used as induction therapy for multiple myeloma (MM) . A meta-analysis suggested that bortezomib-based maintenance therapy improved progression-free survival (PFS) and overall survival (OS) for MM patients . However, it also led to a trend toward increased risk of grade 3 neurologic symptoms, gastrointestinal symptoms, and fatigue .
Chemotherapeutic Drug Interactions
Bortezomib has been shown to have dual effects on fibrosis, with bleomycin exacerbating pulmonary fibrosis and bortezomib alleviating tissue fibrotic processes . Understanding the intricate interplay between chemotherapeutic drugs, immune responses, and pulmonary fibrosis is likely to serve as the foundation for crafting tailored therapeutic strategies .
Immune Response Modulation
In the context of pulmonary fibrosis or TGF-β1 stimulation in vitro, macrophages exhibited an M2-polarized phenotype and secreted more CXCL16 than those of the control group . Bortezomib intervention significantly alleviated fibrosis, and reduced M2 polarization and CXCL16 expression of macrophage . Additionally, bortezomib treatment attenuated the chemotaxis of CXCR6 + CD4 T cells in fibrotic lung tissues .
Mécanisme D'action
Target of Action
Bortezomib, also known as (S)-N-(1-Amino-1-oxo-3-phenylpropan-2-yl)pyrazine-2-carboxamide, primarily targets the 26S proteasome in cells . The 26S proteasome is a protein complex that degrades ubiquitinated proteins in the ubiquitin-proteasome pathway . This pathway plays a crucial role in maintaining protein homeostasis within the cell .
Mode of Action
Bortezomib acts by reversibly inhibiting the chymotrypsin-like activity of the 26S proteasome . This inhibition leads to the accumulation of ubiquitinated proteins, causing disruption in various cellular processes . It prevents the degradation of pro-apoptotic factors, leading to cell cycle arrest and apoptosis of cancer cells .
Biochemical Pathways
The primary biochemical pathway affected by Bortezomib is the ubiquitin-proteasome pathway . By inhibiting the proteasome, Bortezomib disrupts the degradation of intracellular proteins, leading to an accumulation of misfolded proteins . This initiates endoplasmic reticulum (ER) stress and an unfolded protein response (UPR), which play crucial roles in the cytotoxic effect of proteasome inhibitors .
Pharmacokinetics
Bortezomib is metabolized in the liver, with cytochrome P450 (CYP) enzymes playing a significant role in its metabolism . It exhibits a protein binding of approximately 83% . The elimination half-life of Bortezomib ranges from 9 to 15 hours, indicating its relatively short presence in the body .
Result of Action
The molecular and cellular effects of Bortezomib’s action primarily involve the induction of cell cycle arrest and apoptosis . It causes the phosphorylation and cleavage of Bcl-2, a protein that regulates cell death, leading to G2-M phase cell cycle arrest and the induction of apoptosis .
Action Environment
Environmental factors can influence the action, efficacy, and stability of Bortezomib. For instance, certain patient characteristics, such as a low body mass index, can increase the risk of cardiovascular adverse events associated with Bortezomib . Furthermore, the development of drug resistance is a significant challenge in the treatment with Bortezomib .
Propriétés
IUPAC Name |
N-[(2S)-1-amino-1-oxo-3-phenylpropan-2-yl]pyrazine-2-carboxamide | |
---|---|---|
Source | PubChem | |
URL | https://pubchem.ncbi.nlm.nih.gov | |
Description | Data deposited in or computed by PubChem | |
InChI |
InChI=1S/C14H14N4O2/c15-13(19)11(8-10-4-2-1-3-5-10)18-14(20)12-9-16-6-7-17-12/h1-7,9,11H,8H2,(H2,15,19)(H,18,20)/t11-/m0/s1 | |
Source | PubChem | |
URL | https://pubchem.ncbi.nlm.nih.gov | |
Description | Data deposited in or computed by PubChem | |
InChI Key |
KTQJEBWLYWKXRA-NSHDSACASA-N | |
Source | PubChem | |
URL | https://pubchem.ncbi.nlm.nih.gov | |
Description | Data deposited in or computed by PubChem | |
Canonical SMILES |
C1=CC=C(C=C1)CC(C(=O)N)NC(=O)C2=NC=CN=C2 | |
Source | PubChem | |
URL | https://pubchem.ncbi.nlm.nih.gov | |
Description | Data deposited in or computed by PubChem | |
Isomeric SMILES |
C1=CC=C(C=C1)C[C@@H](C(=O)N)NC(=O)C2=NC=CN=C2 | |
Source | PubChem | |
URL | https://pubchem.ncbi.nlm.nih.gov | |
Description | Data deposited in or computed by PubChem | |
Molecular Formula |
C14H14N4O2 | |
Source | PubChem | |
URL | https://pubchem.ncbi.nlm.nih.gov | |
Description | Data deposited in or computed by PubChem | |
DSSTOX Substance ID |
DTXSID80736476 | |
Record name | Nalpha-(Pyrazine-2-carbonyl)-L-phenylalaninamide | |
Source | EPA DSSTox | |
URL | https://comptox.epa.gov/dashboard/DTXSID80736476 | |
Description | DSSTox provides a high quality public chemistry resource for supporting improved predictive toxicology. | |
Molecular Weight |
270.29 g/mol | |
Source | PubChem | |
URL | https://pubchem.ncbi.nlm.nih.gov | |
Description | Data deposited in or computed by PubChem | |
Product Name |
(S)-N-(1-Amino-1-oxo-3-phenylpropan-2-yl)pyrazine-2-carboxamide | |
CAS RN |
289472-80-6 | |
Record name | N-[(1S)-2-Amino-2-oxo-1-(phenylmethyl)ethyl]-2-pyrazinecarboxamide | |
Source | CAS Common Chemistry | |
URL | https://commonchemistry.cas.org/detail?cas_rn=289472-80-6 | |
Description | CAS Common Chemistry is an open community resource for accessing chemical information. Nearly 500,000 chemical substances from CAS REGISTRY cover areas of community interest, including common and frequently regulated chemicals, and those relevant to high school and undergraduate chemistry classes. This chemical information, curated by our expert scientists, is provided in alignment with our mission as a division of the American Chemical Society. | |
Explanation | The data from CAS Common Chemistry is provided under a CC-BY-NC 4.0 license, unless otherwise stated. | |
Record name | N-((1S)-2-Amino-2-oxo-1-(phenylmethyl)ethyl)-pyrazinecarboxamide | |
Source | ChemIDplus | |
URL | https://pubchem.ncbi.nlm.nih.gov/substance/?source=chemidplus&sourceid=0289472806 | |
Description | ChemIDplus is a free, web search system that provides access to the structure and nomenclature authority files used for the identification of chemical substances cited in National Library of Medicine (NLM) databases, including the TOXNET system. | |
Record name | Nalpha-(Pyrazine-2-carbonyl)-L-phenylalaninamide | |
Source | EPA DSSTox | |
URL | https://comptox.epa.gov/dashboard/DTXSID80736476 | |
Description | DSSTox provides a high quality public chemistry resource for supporting improved predictive toxicology. | |
Record name | N-((1S)-2-AMINO-2-OXO-1-(PHENYLMETHYL)ETHYL)-PYRAZINECARBOXAMIDE | |
Source | FDA Global Substance Registration System (GSRS) | |
URL | https://gsrs.ncats.nih.gov/ginas/app/beta/substances/N46QC2PY7V | |
Description | The FDA Global Substance Registration System (GSRS) enables the efficient and accurate exchange of information on what substances are in regulated products. Instead of relying on names, which vary across regulatory domains, countries, and regions, the GSRS knowledge base makes it possible for substances to be defined by standardized, scientific descriptions. | |
Explanation | Unless otherwise noted, the contents of the FDA website (www.fda.gov), both text and graphics, are not copyrighted. They are in the public domain and may be republished, reprinted and otherwise used freely by anyone without the need to obtain permission from FDA. Credit to the U.S. Food and Drug Administration as the source is appreciated but not required. | |
Avertissement et informations sur les produits de recherche in vitro
Veuillez noter que tous les articles et informations sur les produits présentés sur BenchChem sont destinés uniquement à des fins informatives. Les produits disponibles à l'achat sur BenchChem sont spécifiquement conçus pour des études in vitro, qui sont réalisées en dehors des organismes vivants. Les études in vitro, dérivées du terme latin "in verre", impliquent des expériences réalisées dans des environnements de laboratoire contrôlés à l'aide de cellules ou de tissus. Il est important de noter que ces produits ne sont pas classés comme médicaments et n'ont pas reçu l'approbation de la FDA pour la prévention, le traitement ou la guérison de toute condition médicale, affection ou maladie. Nous devons souligner que toute forme d'introduction corporelle de ces produits chez les humains ou les animaux est strictement interdite par la loi. Il est essentiel de respecter ces directives pour assurer la conformité aux normes légales et éthiques en matière de recherche et d'expérimentation.