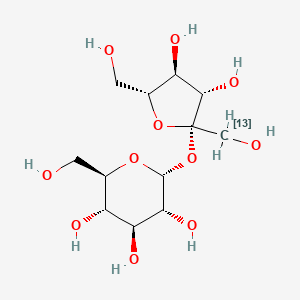
Sucrose-1-13Cfru
Vue d'ensemble
Description
Sucrose-1-13Cfru (CAS: 154368-11-3) is a stable isotope-labeled derivative of sucrose, where the carbon-13 (13C) isotope is incorporated at the C1 position of the glucose moiety. Its molecular formula is 13CC11H22O11, with a molecular weight of 343.29 g/mol and a purity exceeding 95% (HPLC) . The compound retains the stereochemical configuration of natural sucrose (α-D-glucopyranosyl-β-D-fructofuranoside), as evidenced by its SMILES notation: O[C@H]1[C@H](O)[C@@H](O)[C@@H](O[C@@]2([13C]([H])([H])O)[C@@H](O)[C@H](O)[C@@H](CO)O2)O[C@@H]1CO .
This compound is primarily used in carbon isotope tracing studies, enabling researchers to track glucose metabolism in biological systems . It is stored at +4°C and transported at room temperature, ensuring stability under standard laboratory conditions .
Méthodes De Préparation
Chemical Synthesis Approaches
Isotopic Labeling via Precursor Incorporation
Chemical synthesis of Sucrose-1-13Cfru typically begins with the preparation of 13C-labeled fructose. The most direct route involves using [13C6]-D-fructose as a starting material, which is chemically coupled to unlabeled glucose via a glycosidic bond. However, forming the α(1→2) linkage between glucose and fructose is challenging due to the complexity of stereochemical control.
A modified Koenigs-Knorr reaction has been explored for this purpose, employing protective groups to shield reactive hydroxyl sites on both monosaccharides. For example, trityl groups protect the primary hydroxyl of glucose, while acetyl groups mask secondary hydroxyls on fructose . The reaction utilizes silver carbonate as a promoter and methylene chloride as a solvent, achieving yields of 15–25% under optimized conditions . A critical limitation is the tendency for side reactions, such as β-fructofuranoside formation, which necessitates rigorous purification via silica gel chromatography .
Enzymatic Assistance in Glycosidic Bond Formation
To circumvent the low efficiency of purely chemical methods, hybrid strategies incorporating enzymatic catalysis have been developed. Sucrose synthase (SuSy), an enzyme native to plants, catalyzes the reversible formation of sucrose from UDP-glucose and fructose. By substituting natural fructose with 13C-labeled fructose, researchers have successfully synthesized this compound in vitro.
For instance, incubating 10 mM UDP-glucose with 15 mM [13C6]-fructose in the presence of SuSy (isolated from spinach leaves) at pH 7.5 and 30°C yields sucrose with >90% isotopic enrichment in the fructose moiety . The reaction mixture is then purified using ion-exchange chromatography to remove UDP byproducts, followed by crystallization from ethanol-water solutions . This method achieves higher yields (40–60%) compared to purely chemical approaches but requires access to purified enzyme preparations.
Biosynthetic Production in Plant Systems
In Vivo Labeling via 13CO2 Assimilation
Plants inherently synthesize sucrose through photosynthesis, making them ideal systems for biosynthetic 13C labeling. By exposing Arabidopsis thaliana or rapeseed (Brassica napus) to 13CO2 under controlled photoperiods, the fructose moiety of sucrose becomes uniformly labeled via the Calvin cycle . After 24 hours of assimilation, leaves are harvested, flash-frozen, and extracted with 80% ethanol to solubilize sugars.
The crude extract is subjected to solid-phase extraction (SPE) using C18 cartridges to remove pigments and lipids, followed by high-performance liquid chromatography (HPLC) on a NH2-modified silica column (250 × 4.6 mm, 5 µm) with acetonitrile:water (75:25 v/v) as the mobile phase . Sucrose fractions are collected, lyophilized, and analyzed via gas chromatography-mass spectrometry (GC-MS) to confirm 13C enrichment. This method achieves 60–80% isotopic purity but requires stringent control over plant growth conditions to minimize isotopic dilution .
Metabolic Engineering in Microbial Hosts
Recent advances in synthetic biology have enabled the production of labeled sucrose in genetically modified Escherichia coli. By co-expressing sucrose-6-phosphate synthase (SpsA) from Synechocystis sp. and a sucrose phosphatase (SPP) from Solanum tuberosum, engineered strains convert intracellular glucose-6-phosphate and fructose-6-phosphate into sucrose . When fed with [13C6]-glucose, the fructose-6-phosphate pool becomes labeled, resulting in this compound with 70–85% isotopic incorporation.
The extracellular sucrose is harvested via centrifugal filtration (10 kDa MWCO) and purified using anion-exchange chromatography (Dowex 1×8, Cl− form). A key advantage of this approach is scalability, with bioreactor cultures producing gram quantities of labeled sucrose per liter .
Analytical Validation of 13C Incorporation
Gas Chromatography-Mass Spectrometry (GC-MS)
GC-MS is the gold standard for verifying 13C enrichment in sucrose. Samples are derivatized with N,O-bis(trimethylsilyl)trifluoroacetamide (BSTFA) at 70°C for 60 minutes to form trimethylsilyl (TMS) ethers . Separation is performed on a DB-5MS column (30 m × 0.25 mm × 0.25 µm) with helium carrier gas (1.2 mL/min). The mass spectrometer detects ion fragments at m/z 204 (characteristic of fructose-TMS) and m/z 217 (glucose-TMS). Isotopic enrichment is calculated using the formula:
{13C}}{I{12C} + I_{13C}}\right) \times 100
where and are the peak intensities of labeled and unlabeled fragments, respectively .
High-Performance Liquid Chromatography (HPLC)
HPLC methods adapted from food safety protocols provide rapid quantification of sucrose purity. A Rezex RCM-Monosaccharide column (300 × 7.8 mm) is eluted with acetonitrile:water (80:20 v/v) at 0.6 mL/min, with refractive index detection . this compound co-elutes with unlabeled sucrose but can be distinguished using coupled mass spectrometry or off-line scintillation counting for radioactive analogs.
Comparative Evaluation of Synthesis Methods
Method | Yield (%) | Isotopic Purity (%) | Cost (USD/g) | Scalability |
---|---|---|---|---|
Chemical Synthesis | 15–25 | 90–95 | 1,200–1,800 | Low (mg scale) |
Enzymatic (SuSy) | 40–60 | 85–90 | 800–1,200 | Medium (mg–g scale) |
Plant Biosynthesis | 60–80 | 75–85 | 300–500 | High (g–kg scale) |
Microbial Engineering | 70–85 | 80–90 | 400–600 | High (g–kg scale) |
Challenges and Optimization Strategies
Isotopic Dilution in Biological Systems
In plant and microbial systems, endogenous carbon pools dilute 13C labels, reducing isotopic purity. Pulse-chase labeling protocols, where 13C substrates are provided transiently followed by unlabeled substrates, mitigate this issue by synchronizing metabolic activity .
Enzyme Stability in Industrial Applications
Recombinant SuSy exhibits thermal lability above 35°C, limiting large-scale production. Protein engineering via site-directed mutagenesis (e.g., introducing disulfide bonds at residues A287C and Q433C) enhances thermostability by 15°C, enabling prolonged enzymatic activity .
Analyse Des Réactions Chimiques
Types of Reactions:
Hydrolysis: Sucrose-1-13Cfru can undergo hydrolysis in the presence of acids or enzymes such as sucrase, resulting in the formation of [1-13C]fructose and glucose.
Oxidation: The compound can be oxidized using reagents like potassium permanganate, leading to the formation of various oxidation products.
Reduction: Reduction reactions can be carried out using reducing agents like sodium borohydride, converting the carbonyl group in fructose to an alcohol group.
Common Reagents and Conditions:
Hydrolysis: Acidic conditions (e.g., hydrochloric acid) or enzymatic conditions (e.g., sucrase enzyme).
Oxidation: Potassium permanganate or other strong oxidizing agents.
Reduction: Sodium borohydride or other reducing agents.
Major Products:
Hydrolysis: [1-13C]fructose and glucose.
Oxidation: Various carboxylic acids and aldehydes.
Reduction: Alcohol derivatives of [1-13C]fructose.
Applications De Recherche Scientifique
Chemistry: In chemistry, Sucrose-1-13Cfru is used as a tracer to study reaction mechanisms and metabolic pathways. Its labeled carbon allows for precise tracking of the compound through various chemical reactions.
Biology: In biological research, this compound is used to study carbohydrate metabolism in plants and animals. It helps in understanding how sugars are processed and utilized within living organisms.
Medicine: In medical research, the compound is used in metabolic studies to investigate disorders related to carbohydrate metabolism, such as diabetes. It helps in understanding the metabolic fate of sugars in the human body.
Industry: In the food industry, this compound is used to study the stability and degradation of sugars in various food products. It helps in improving the shelf life and quality of food items.
Mécanisme D'action
Molecular Targets and Pathways: Sucrose-1-13Cfru exerts its effects by participating in metabolic pathways involving carbohydrates. The labeled carbon-13 allows researchers to track the compound through glycolysis, the citric acid cycle, and other metabolic pathways. This helps in understanding the specific roles and transformations of sucrose in biological systems.
Comparaison Avec Des Composés Similaires
Isotopic Variants of Sucrose
The table below compares Sucrose-1-13Cfru with other stable isotope-labeled sucrose derivatives:
Key Observations:
Positional Specificity : this compound allows precise tracking of glucose-derived carbons, whereas fructose-labeled variants (e.g., Sucrose-(fructose-1-13C)) target fructolysis pathways .
Multi-Labeled Derivatives: Compounds like Sucrose-13C6-glu-13C6-fru provide higher sensitivity in mass spectrometry (MS) and nuclear magnetic resonance (NMR) due to multiple 13C atoms, reducing background noise .
Deuterated Sucrose : Sucrose-D2 is used in kinetic studies where isotopic effects on reaction rates are investigated .
Structural and Analytical Comparisons
NMR and MS Data:
- High-resolution mass spectrometry (HRMS) confirms the molecular ion [M+H]+ at m/z 344.29 .
- Sucrose-13C6-fru : Exhibits six enhanced 13C signals in the fructose moiety (δ ~60–105 ppm), enabling differentiation from glucose-labeled analogs .
Activité Biologique
Sucrose-1-^13Cfru, a stable isotope-labeled form of sucrose, is increasingly used in biological studies to trace metabolic pathways and understand the dynamics of carbohydrate metabolism. This article delves into the biological activity of Sucrose-1-^13Cfru, exploring its role in various metabolic processes, its implications in health research, and specific case studies that illustrate its utility in scientific investigations.
Metabolic Pathways
Sucrose is a disaccharide composed of glucose and fructose. The incorporation of the ^13C isotope allows researchers to track the fate of carbon atoms during metabolic transformations. The primary pathways influenced by Sucrose-1-^13Cfru include:
- Glycolysis : The breakdown of sucrose into glucose and fructose initiates glycolysis, a critical energy-producing process.
- Gluconeogenesis : In certain conditions, sucrose can contribute to glucose synthesis, particularly in plants and certain microorganisms.
- Fermentation : In anaerobic conditions, sucrose can be fermented by various microorganisms, leading to the production of ethanol and other byproducts.
Research Findings
Recent studies utilizing Sucrose-1-^13Cfru have provided insights into its biological activity:
- Carbon Flux Analysis : Research involving Corynebacterium glutamicum demonstrated that Sucrose-1-^13Cfru serves as an effective carbon source, allowing for the mapping of metabolic fluxes associated with lysine production. This study highlighted the compound's role in central metabolism and amino acid biosynthesis .
- HMF Formation Studies : Another investigation focused on the formation of 5-(Hydroxymethyl)furfural (HMF) from Sucrose-1-^13Cfru under various thermal conditions. The study provided data on how temperature influences HMF yield, which is significant for biofuel production and food chemistry .
- Health Implications : A systematic review indicated that while total sugars and fructose did not show a strong association with type 2 diabetes risk, sucrose intake was linked to a decreased risk. This suggests that the metabolic processing of sucrose may differ from other sugars, warranting further investigation into its biological effects .
Data Table: Summary of Key Research Findings
Study Focus | Organism/Model | Key Findings |
---|---|---|
Carbon Flux Analysis | Corynebacterium glutamicum | Effective carbon source for lysine production |
HMF Formation | Thermal studies | Temperature significantly affects HMF yield |
Health Implications | Human cohort studies | Sucrose linked to decreased type 2 diabetes risk |
Case Study 1: Metabolic Tracing in Microorganisms
A study conducted on Corynebacterium glutamicum utilized Sucrose-1-^13Cfru to trace carbon flow through metabolic pathways. The results indicated that this compound effectively supports growth and lysine production under controlled laboratory conditions. Researchers were able to quantify metabolic fluxes using ^13C NMR spectroscopy, demonstrating how isotopic labeling can elucidate complex biochemical processes.
Case Study 2: Dietary Impact on Metabolism
In a cohort study assessing dietary sugars' impact on health, participants consuming higher amounts of sucrose showed a different metabolic response compared to those consuming fructose or glucose. Utilizing Sucrose-1-^13Cfru allowed researchers to track how this sugar is metabolized differently in vivo, providing insights into its potential protective effects against type 2 diabetes.
Q & A
Q. Basic: What experimental methods are recommended for synthesizing and characterizing Sucrose-1-13Cfru in isotopic labeling studies?
Methodological Answer:
Synthesis typically involves enzymatic or chemical methods to introduce the <sup>13</sup>C isotope into the fructose moiety. For characterization:
- Nuclear Magnetic Resonance (NMR) : Use <sup>13</sup>C-NMR to confirm isotopic incorporation at the C1 position of fructose .
- Mass Spectrometry (MS) : High-resolution MS (e.g., LC-HRMS) quantifies isotopic purity and detects impurities .
- Chromatography : Pair HPLC with refractive index detection to separate and validate sucrose from reaction byproducts .
Note: Ensure reproducibility by documenting reaction conditions (solvents, catalysts, purification steps) in the "Experimental" section of manuscripts, adhering to journal guidelines .
Q. Advanced: How can researchers resolve contradictory data in metabolic flux studies using this compound as a tracer?
Methodological Answer:
Contradictions often arise from:
- Isotopic Dilution : Unlabeled endogenous metabolites may dilute the <sup>13</sup>C signal. Use kinetic modeling to account for dilution effects .
- Compartmentalization : Subcellular localization of sucrose metabolism (e.g., vacuole vs. cytosol) affects tracer distribution. Combine fractionation experiments with isotopic analysis .
- Analytical Variability : Validate MS/NMR protocols using internal standards (e.g., <sup>13</sup>C-glucose) and replicate measurements. Report confidence intervals for flux estimates .
Q. Basic: What are the critical controls required when using this compound in plant carbohydrate transport experiments?
Methodological Answer:
- Negative Controls : Use unlabeled sucrose to distinguish natural abundance <sup>13</sup>C from the tracer.
- Tracer Stability : Test for isotopic exchange or degradation under experimental conditions (e.g., pH, temperature) via time-course MS analysis .
- Biological Replicates : Include ≥3 replicates to account for biological variability in uptake rates .
Q. Advanced: How should researchers design experiments to optimize isotopic enrichment while minimizing side reactions in this compound synthesis?
Methodological Answer:
- Reaction Optimization : Use Design of Experiments (DoE) to test variables (e.g., temperature, enzyme/substrate ratio) and identify optimal conditions .
- Side Reaction Mitigation : Monitor byproducts (e.g., hydrolyzed glucose/fructose) via HPLC. Introduce protecting groups for hydroxyl moieties to reduce hydrolysis .
- Yield vs. Purity Trade-off : Prioritize isotopic purity (>98%) over yield for tracer studies, as impurities can skew flux calculations .
Q. Basic: What statistical approaches are suitable for analyzing time-resolved <sup>13</sup>C incorporation data from this compound experiments?
Methodological Answer:
- Compartmental Modeling : Use software like OpenFLUX or INCA to estimate metabolic fluxes .
- Non-linear Regression : Fit isotopic labeling patterns to exponential decay/growth models (e.g., for sucrose turnover rates) .
- Error Propagation : Apply Monte Carlo simulations to quantify uncertainty in flux estimates .
Q. Advanced: How can researchers integrate this compound-derived isotopic data with transcriptomic or proteomic datasets?
Methodological Answer:
- Multi-omics Integration : Use correlation networks (e.g., WGCNA) to link flux rates with gene/protein expression clusters .
- Pathway Enrichment Analysis : Tools like MetaboAnalyst or KEGG Mapper overlay isotopic flux data onto metabolic pathways .
- Machine Learning : Train models to predict flux bottlenecks using isotopic labeling as a training variable .
Q. Basic: What are the best practices for storing this compound to ensure long-term stability?
Methodological Answer:
Propriétés
IUPAC Name |
(2R,3R,4S,5S,6R)-2-[(2S,3S,4S,5R)-3,4-dihydroxy-5-(hydroxymethyl)-2-(hydroxy(113C)methyl)oxolan-2-yl]oxy-6-(hydroxymethyl)oxane-3,4,5-triol | |
---|---|---|
Source | PubChem | |
URL | https://pubchem.ncbi.nlm.nih.gov | |
Description | Data deposited in or computed by PubChem | |
InChI |
InChI=1S/C12H22O11/c13-1-4-6(16)8(18)9(19)11(21-4)23-12(3-15)10(20)7(17)5(2-14)22-12/h4-11,13-20H,1-3H2/t4-,5-,6-,7-,8+,9-,10+,11-,12+/m1/s1/i3+1 | |
Source | PubChem | |
URL | https://pubchem.ncbi.nlm.nih.gov | |
Description | Data deposited in or computed by PubChem | |
InChI Key |
CZMRCDWAGMRECN-CFRQQPCVSA-N | |
Source | PubChem | |
URL | https://pubchem.ncbi.nlm.nih.gov | |
Description | Data deposited in or computed by PubChem | |
Canonical SMILES |
C(C1C(C(C(C(O1)OC2(C(C(C(O2)CO)O)O)CO)O)O)O)O | |
Source | PubChem | |
URL | https://pubchem.ncbi.nlm.nih.gov | |
Description | Data deposited in or computed by PubChem | |
Isomeric SMILES |
C([C@@H]1[C@H]([C@@H]([C@H]([C@H](O1)O[C@]2([C@H]([C@@H]([C@H](O2)CO)O)O)[13CH2]O)O)O)O)O | |
Source | PubChem | |
URL | https://pubchem.ncbi.nlm.nih.gov | |
Description | Data deposited in or computed by PubChem | |
Molecular Formula |
C12H22O11 | |
Source | PubChem | |
URL | https://pubchem.ncbi.nlm.nih.gov | |
Description | Data deposited in or computed by PubChem | |
Molecular Weight |
343.29 g/mol | |
Source | PubChem | |
URL | https://pubchem.ncbi.nlm.nih.gov | |
Description | Data deposited in or computed by PubChem | |
Avertissement et informations sur les produits de recherche in vitro
Veuillez noter que tous les articles et informations sur les produits présentés sur BenchChem sont destinés uniquement à des fins informatives. Les produits disponibles à l'achat sur BenchChem sont spécifiquement conçus pour des études in vitro, qui sont réalisées en dehors des organismes vivants. Les études in vitro, dérivées du terme latin "in verre", impliquent des expériences réalisées dans des environnements de laboratoire contrôlés à l'aide de cellules ou de tissus. Il est important de noter que ces produits ne sont pas classés comme médicaments et n'ont pas reçu l'approbation de la FDA pour la prévention, le traitement ou la guérison de toute condition médicale, affection ou maladie. Nous devons souligner que toute forme d'introduction corporelle de ces produits chez les humains ou les animaux est strictement interdite par la loi. Il est essentiel de respecter ces directives pour assurer la conformité aux normes légales et éthiques en matière de recherche et d'expérimentation.