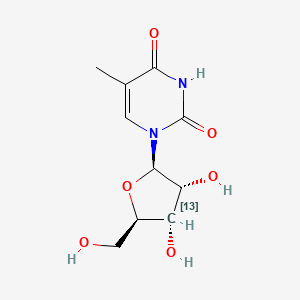
5-Methyluridine-3'-13C
- Cliquez sur DEMANDE RAPIDE pour recevoir un devis de notre équipe d'experts.
- Avec des produits de qualité à un prix COMPÉTITIF, vous pouvez vous concentrer davantage sur votre recherche.
Vue d'ensemble
Description
5-Methyl-[3’-13C]uridine is a labeled nucleoside analog of 5-methyluridine, where the carbon-13 isotope is incorporated at the 3’ position of the ribose sugar. This compound is a pyrimidine nucleoside, which means it contains a pyrimidine base (thymine) attached to a ribose sugar. The labeling with carbon-13 makes it useful in various scientific studies, particularly in nuclear magnetic resonance (NMR) spectroscopy and metabolic studies.
Méthodes De Préparation
Synthetic Routes and Reaction Conditions
The synthesis of 5-Methyl-[3’-13C]uridine typically involves the incorporation of the carbon-13 isotope into the ribose sugar, followed by the attachment of the thymine base. One common method involves the use of labeled ribose precursors, which are then chemically modified to form the desired nucleoside. The reaction conditions often include the use of protecting groups to ensure selective reactions at specific positions on the ribose and thymine molecules.
Industrial Production Methods
Industrial production of 5-Methyl-[3’-13C]uridine may involve large-scale synthesis using similar methods as described above, but with optimizations for yield and purity. This can include the use of automated synthesizers and high-performance liquid chromatography (HPLC) for purification.
Analyse Des Réactions Chimiques
Types of Reactions
5-Methyl-[3’-13C]uridine can undergo various chemical reactions, including:
Oxidation: The hydroxyl groups on the ribose sugar can be oxidized to form ketones or aldehydes.
Reduction: The carbonyl groups can be reduced to form alcohols.
Substitution: The hydroxyl groups can be substituted with other functional groups, such as halogens or alkyl groups.
Common Reagents and Conditions
Common reagents used in these reactions include oxidizing agents like potassium permanganate or chromium trioxide, reducing agents like sodium borohydride or lithium aluminum hydride, and substitution reagents like halogenating agents or alkyl halides. The reaction conditions typically involve controlled temperatures and pH levels to ensure selective reactions.
Major Products
The major products formed from these reactions depend on the specific reagents and conditions used. For example, oxidation of the hydroxyl groups can lead to the formation of ribose derivatives with ketone or aldehyde functional groups, while substitution reactions can yield halogenated or alkylated nucleosides.
Applications De Recherche Scientifique
5-Methyl-[3’-13C]uridine has a wide range of scientific research applications, including:
Chemistry: Used as a labeled compound in NMR spectroscopy to study the structure and dynamics of nucleic acids and other biomolecules.
Biology: Employed in metabolic studies to trace the incorporation and metabolism of nucleosides in cells.
Medicine: Investigated for its potential role in cancer research, particularly in studying the metabolism of nucleosides in cancer cells.
Industry: Utilized in the development of diagnostic tools and therapeutic agents, particularly in the field of personalized medicine.
Mécanisme D'action
The mechanism of action of 5-Methyl-[3’-13C]uridine involves its incorporation into nucleic acids, where it can affect the stability and function of RNA and DNA molecules. The carbon-13 label allows researchers to track its incorporation and metabolism using NMR spectroscopy. The molecular targets and pathways involved include various enzymes responsible for nucleoside metabolism, such as nucleoside phosphorylases and kinases.
Comparaison Avec Des Composés Similaires
Similar Compounds
5-Methyluridine: The non-labeled counterpart of 5-Methyl-[3’-13C]uridine, commonly found in tRNA and other RNA molecules.
3-Methyluridine: Another methylated nucleoside with a methyl group at the 3-position of the uracil base.
5-Methylcytosine: A methylated cytosine base found in DNA, involved in epigenetic regulation.
Uniqueness
The uniqueness of 5-Methyl-[3’-13C]uridine lies in its carbon-13 labeling, which makes it particularly useful for NMR spectroscopy and metabolic studies. This labeling allows for precise tracking and analysis of its incorporation and metabolism in biological systems, providing valuable insights into nucleoside dynamics and function.
Propriétés
IUPAC Name |
1-[(2R,3R,4S,5R)-3,4-dihydroxy-5-(hydroxymethyl)(413C)oxolan-2-yl]-5-methylpyrimidine-2,4-dione |
Source
|
---|---|---|
Source | PubChem | |
URL | https://pubchem.ncbi.nlm.nih.gov | |
Description | Data deposited in or computed by PubChem | |
InChI |
InChI=1S/C10H14N2O6/c1-4-2-12(10(17)11-8(4)16)9-7(15)6(14)5(3-13)18-9/h2,5-7,9,13-15H,3H2,1H3,(H,11,16,17)/t5-,6-,7-,9-/m1/s1/i6+1 |
Source
|
Source | PubChem | |
URL | https://pubchem.ncbi.nlm.nih.gov | |
Description | Data deposited in or computed by PubChem | |
InChI Key |
DWRXFEITVBNRMK-KHDHUMRTSA-N |
Source
|
Source | PubChem | |
URL | https://pubchem.ncbi.nlm.nih.gov | |
Description | Data deposited in or computed by PubChem | |
Canonical SMILES |
CC1=CN(C(=O)NC1=O)C2C(C(C(O2)CO)O)O |
Source
|
Source | PubChem | |
URL | https://pubchem.ncbi.nlm.nih.gov | |
Description | Data deposited in or computed by PubChem | |
Isomeric SMILES |
CC1=CN(C(=O)NC1=O)[C@H]2[C@@H]([13C@@H]([C@H](O2)CO)O)O |
Source
|
Source | PubChem | |
URL | https://pubchem.ncbi.nlm.nih.gov | |
Description | Data deposited in or computed by PubChem | |
Molecular Formula |
C10H14N2O6 |
Source
|
Source | PubChem | |
URL | https://pubchem.ncbi.nlm.nih.gov | |
Description | Data deposited in or computed by PubChem | |
Molecular Weight |
259.22 g/mol |
Source
|
Source | PubChem | |
URL | https://pubchem.ncbi.nlm.nih.gov | |
Description | Data deposited in or computed by PubChem | |
Avertissement et informations sur les produits de recherche in vitro
Veuillez noter que tous les articles et informations sur les produits présentés sur BenchChem sont destinés uniquement à des fins informatives. Les produits disponibles à l'achat sur BenchChem sont spécifiquement conçus pour des études in vitro, qui sont réalisées en dehors des organismes vivants. Les études in vitro, dérivées du terme latin "in verre", impliquent des expériences réalisées dans des environnements de laboratoire contrôlés à l'aide de cellules ou de tissus. Il est important de noter que ces produits ne sont pas classés comme médicaments et n'ont pas reçu l'approbation de la FDA pour la prévention, le traitement ou la guérison de toute condition médicale, affection ou maladie. Nous devons souligner que toute forme d'introduction corporelle de ces produits chez les humains ou les animaux est strictement interdite par la loi. Il est essentiel de respecter ces directives pour assurer la conformité aux normes légales et éthiques en matière de recherche et d'expérimentation.