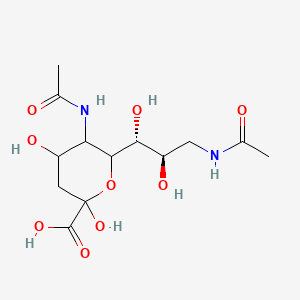
N-Acetyl-9-(acetylamino)-9-deoxyneuraminic acid
- Cliquez sur DEMANDE RAPIDE pour recevoir un devis de notre équipe d'experts.
- Avec des produits de qualité à un prix COMPÉTITIF, vous pouvez vous concentrer davantage sur votre recherche.
Vue d'ensemble
Description
N-Acetyl-9-(acetylamino)-9-deoxyneuraminic acid: is a complex organic compound with significant applications in various scientific fields. This compound is characterized by its intricate structure, which includes multiple hydroxyl groups, acetamido groups, and a carboxylic acid group. It is often studied for its potential biological and chemical properties.
Méthodes De Préparation
Synthetic Routes and Reaction Conditions
The synthesis of N-Acetyl-9-(acetylamino)-9-deoxyneuraminic acid typically involves multiple steps, including the protection and deprotection of functional groups, as well as selective acylation and hydroxylation reactions. One common synthetic route involves the following steps:
Protection of Hydroxyl Groups: The hydroxyl groups are protected using silyl or benzyl protecting groups to prevent unwanted reactions.
Acylation: The protected intermediate is then subjected to acylation reactions to introduce the acetamido groups.
Deprotection: The protecting groups are removed to yield the final compound.
Industrial Production Methods
Industrial production of this compound may involve similar synthetic routes but on a larger scale. The use of automated reactors and continuous flow systems can enhance the efficiency and yield of the synthesis. Additionally, purification techniques such as crystallization and chromatography are employed to obtain the compound in high purity.
Analyse Des Réactions Chimiques
Types of Reactions
N-Acetyl-9-(acetylamino)-9-deoxyneuraminic acid undergoes various chemical reactions, including:
Oxidation: The hydroxyl groups can be oxidized to form ketones or aldehydes.
Reduction: The compound can be reduced to form alcohols or amines.
Substitution: The acetamido groups can be substituted with other functional groups using nucleophilic substitution reactions.
Common Reagents and Conditions
Oxidation: Reagents such as potassium permanganate (KMnO4) or chromium trioxide (CrO3) are commonly used.
Reduction: Sodium borohydride (NaBH4) or lithium aluminum hydride (LiAlH4) are typical reducing agents.
Substitution: Nucleophiles such as amines or thiols can be used under basic conditions.
Major Products
The major products formed from these reactions depend on the specific conditions and reagents used. For example, oxidation can yield ketones or aldehydes, while reduction can produce alcohols or amines.
Applications De Recherche Scientifique
N-Acetyl-9-(acetylamino)-9-deoxyneuraminic acid has a wide range of applications in scientific research:
Chemistry: It is used as a building block in the synthesis of more complex molecules.
Biology: The compound is studied for its potential biological activity, including antimicrobial and antiviral properties.
Medicine: It is investigated for its potential therapeutic applications, such as in the development of new drugs.
Industry: The compound is used in the production of specialty chemicals and materials.
Mécanisme D'action
The mechanism of action of N-Acetyl-9-(acetylamino)-9-deoxyneuraminic acid involves its interaction with specific molecular targets and pathways. The acetamido groups can form hydrogen bonds with biological molecules, while the hydroxyl and carboxylic acid groups can participate in various biochemical reactions. These interactions can modulate the activity of enzymes and receptors, leading to the compound’s observed effects.
Comparaison Avec Des Composés Similaires
Similar Compounds
- (6R)-5-Acetamido-6-[(1R,2R)-3-acetoxy-1,2-dihydroxypropyl]-3,5-dideoxy-4-O-α-D-glucopyranosyl-β-L-threo-hex-2-ulopyranosonate
- (2S,4S,5R,6R)-5-Acetamido-6-[(1S,2R)-2-[(2S,4S,5R,6R)-5-acetamido-2-carboxy-4-hydroxy-6-[(1R,2R)-1,2,3-trihydroxypropyl]oxan-2-yl]oxy-1,3-dihydroxypropyl]-2-[(2S,3R,4S,5S,6R)-2-[(2R,3S,4R,5R,6S)-5-acetamido-6-[(2R,3S,4S,5R,6S)-3,5-dihydroxy-2-(hydroxymethyl)-6-[(2R,3S,4R,5R,6R)-4,5,6-trihydroxy-2-(hydroxymethyl)oxan-3-yl]oxyoxan-4-yl]oxy-4-hydroxy-2-(hydroxymethyl)oxan-3-yl]oxy-3,5-dihydroxy-6-(hydroxymethyl)oxan-4-yl]oxy-4-hydroxyoxane-2-carboxylic acid
Uniqueness
The uniqueness of N-Acetyl-9-(acetylamino)-9-deoxyneuraminic acid lies in its specific arrangement of functional groups, which imparts distinct chemical and biological properties. Its ability to form multiple hydrogen bonds and participate in various biochemical reactions makes it a valuable compound for research and industrial applications.
Activité Biologique
N-Acetyl-9-(acetylamino)-9-deoxyneuraminic acid (commonly referred to as NANA) is a derivative of neuraminic acid, classified as an O-acetylated sialic acid. Its unique structure, characterized by an acetylamino group at the 9-position, enhances its biological activity and specificity in interactions compared to other sialic acids. This article explores the biological activities of NANA, focusing on its roles in cell signaling, immune response modulation, and interactions with pathogens.
- Molecular Formula : C₁₃H₂₂N₂O₉
- Molecular Weight : Approximately 350.32 g/mol
Biological Roles
NANA plays several critical roles in biological systems:
- Cell Signaling : NANA is involved in cell-to-cell communication and recognition processes due to its presence on glycoproteins and glycolipids. It acts as a ligand for various receptors, facilitating cellular interactions essential for normal physiological functions .
- Immune Response Modulation : The compound has been shown to interact with immunoinhibitory sialic acid-binding immunoglobulin-like lectins (Siglecs), which are crucial for preventing autoimmunity and modulating immune responses .
- Pathogen Interaction : NANA serves as a receptor determinant for several viruses, including influenza and bovine coronavirus (BCV). Its ability to bind specifically to viral hemagglutinins allows it to facilitate viral entry into host cells .
The biological activity of NANA is largely dependent on its specific location within glycoconjugates. The following mechanisms have been identified:
- Viral Entry Facilitation : Studies indicate that NANA binds to influenza virus hemagglutinin, which is essential for the virus's entry into host cells. This interaction underscores the potential of NANA in developing antiviral therapies .
- Cell Surface Receptor Functionality : NANA's presence on cell surfaces enhances the recognition of cells by pathogens, thereby influencing infection dynamics. For example, BCV utilizes 9-O-acetylated sialic acid as a receptor determinant for infection in cultured cells .
Comparative Analysis with Other Sialic Acids
The structural uniqueness of NANA allows it to exhibit distinct biological activities compared to other sialic acids. The following table summarizes key differences:
Compound Name | Structure Characteristics | Unique Features |
---|---|---|
N-Acetylneuraminic Acid (Neu5Ac) | Basic sialic acid structure without acetyl groups | Precursor to many sialic acids; lacks additional modifications |
9-Amino-N-acetylneuraminic Acid | Contains an amino group instead of acetyl at position 9 | Involved in different biological pathways |
N-Acetyl-9-O-acetylneuraminic Acid | O-acetylated at position 9 but lacks acetylamino group | Primarily involved in influenza virus interactions |
N-Acetyl-9-azido-9-deoxy-neuraminic Acid | Contains an azido group instead of acetyl | Used for metabolic labeling studies |
Interaction with Influenza Virus
Research has demonstrated that NANA binds to the hemagglutinin protein on the surface of influenza viruses, facilitating their entry into host cells. This interaction highlights the potential for targeting NANA in antiviral drug development .
Immune Evasion by Pathogens
Certain pathogenic bacteria have evolved mechanisms to express sialic acids like NANA on their surfaces, allowing them to evade host immune responses. For instance, Group B Streptococcus presents terminal α2,3-linked sialic acid on its capsular polysaccharide, effectively blocking immune activation by binding Siglecs expressed by immune cells .
Research Findings
Recent studies emphasize the importance of NANA in various biological processes:
- Cellular Development : Sialic acids, including NANA, are crucial for neuronal development and function. They influence cell signaling pathways that regulate neuronal cell behavior .
- Therapeutics Potential : Due to their role in immune modulation and pathogen interaction, sialic acids are being explored for therapeutic applications in treating infections and autoimmune diseases .
Propriétés
IUPAC Name |
5-acetamido-6-[(1R,2R)-3-acetamido-1,2-dihydroxypropyl]-2,4-dihydroxyoxane-2-carboxylic acid |
Source
|
---|---|---|
Source | PubChem | |
URL | https://pubchem.ncbi.nlm.nih.gov | |
Description | Data deposited in or computed by PubChem | |
InChI |
InChI=1S/C13H22N2O9/c1-5(16)14-4-8(19)10(20)11-9(15-6(2)17)7(18)3-13(23,24-11)12(21)22/h7-11,18-20,23H,3-4H2,1-2H3,(H,14,16)(H,15,17)(H,21,22)/t7?,8-,9?,10-,11?,13?/m1/s1 |
Source
|
Source | PubChem | |
URL | https://pubchem.ncbi.nlm.nih.gov | |
Description | Data deposited in or computed by PubChem | |
InChI Key |
VOVFDZJABRGWTQ-WVPFUJCWSA-N |
Source
|
Source | PubChem | |
URL | https://pubchem.ncbi.nlm.nih.gov | |
Description | Data deposited in or computed by PubChem | |
Canonical SMILES |
CC(=O)NCC(C(C1C(C(CC(O1)(C(=O)O)O)O)NC(=O)C)O)O |
Source
|
Source | PubChem | |
URL | https://pubchem.ncbi.nlm.nih.gov | |
Description | Data deposited in or computed by PubChem | |
Isomeric SMILES |
CC(=O)NC[C@H]([C@H](C1C(C(CC(O1)(C(=O)O)O)O)NC(=O)C)O)O |
Source
|
Source | PubChem | |
URL | https://pubchem.ncbi.nlm.nih.gov | |
Description | Data deposited in or computed by PubChem | |
Molecular Formula |
C13H22N2O9 |
Source
|
Source | PubChem | |
URL | https://pubchem.ncbi.nlm.nih.gov | |
Description | Data deposited in or computed by PubChem | |
Molecular Weight |
350.32 g/mol |
Source
|
Source | PubChem | |
URL | https://pubchem.ncbi.nlm.nih.gov | |
Description | Data deposited in or computed by PubChem | |
Avertissement et informations sur les produits de recherche in vitro
Veuillez noter que tous les articles et informations sur les produits présentés sur BenchChem sont destinés uniquement à des fins informatives. Les produits disponibles à l'achat sur BenchChem sont spécifiquement conçus pour des études in vitro, qui sont réalisées en dehors des organismes vivants. Les études in vitro, dérivées du terme latin "in verre", impliquent des expériences réalisées dans des environnements de laboratoire contrôlés à l'aide de cellules ou de tissus. Il est important de noter que ces produits ne sont pas classés comme médicaments et n'ont pas reçu l'approbation de la FDA pour la prévention, le traitement ou la guérison de toute condition médicale, affection ou maladie. Nous devons souligner que toute forme d'introduction corporelle de ces produits chez les humains ou les animaux est strictement interdite par la loi. Il est essentiel de respecter ces directives pour assurer la conformité aux normes légales et éthiques en matière de recherche et d'expérimentation.